Iron and Steel
In 2023, the iron and steel industry generated around 3.49 billion tonnes of CO2 equivalent (CO2e) emissions, representing approximately 5.71% of the global 61.15 billion tonnes of emissions (Source: Climate TRACE).
Primary steelmaking involves several stages. First, the raw materials, mainly iron ore, are extracted. Next, the iron ore is processed to form sintered ore and pellets. These materials, along with other inputs such as coke and limestone/lime, are fed into a blast furnace to produce pig iron. The liquid pig iron is then further processed in a Basic Oxygen Furnace (BOF), where pure oxygen is blown into the molten metal to reduce its carbon content and produce crude steel. This crude steel is finally transformed into finished steel products through multiple subsequent steps.
In 2023, the Blast Furnace-Basic Oxygen Furnace (BF-BOF) route accounted for more than two-thirds (nearly 72%) of global steel production capacity. However, steel is also produced via alternative industrial routes. One such method is the Direct Reduced Iron–Electric Arc Furnace (DRI-EAF) route, which generates lower emissions.
While iron ore constitutes the majority of metallic input in primary steelmaking, the remaining input comes from scrap, which yields secondary (scrap-based) steel. Secondary steel is produced in Electric Arc Furnaces and is generally associated with lower CO₂ emission levels than steel produced via the BF-BOF or DRI-EAF routes.
Integrated steel mills are facilities where the complete production process, from raw materials to finished products, occurs in one location. These facilities typically include sinter plants, coke ovens (for processing coking coal into coke), blast furnaces, steelmaking shops, and rolling mills.
What are the main CO2 emission sources in the iron and steel industry?
Multiple stages generate CO₂ emissions in iron and steel processes, and they are related to three main factors:
a) Providing high temperatures needed to carry out the chemical reactions and physical treatment needed;
b) Providing a reductant (mainly carbon monoxide, CO) to reduce iron oxide to iron. The resulting CO₂ emissions depend largely on the types and amounts of reducing agents used;
c) Providing power and heat (steam) necessary to run the steelworks.
1️⃣ EU CBAM Reminder: If you operate a steel mill, you should use the mass balance method to calculate emissions. Carbon remaining in the product or in slags or wastes (e.g., waste gases) is taken into account using this method.
2️⃣ EU CBAM Reminder: Coke is not a CBAM good. As such, it is treated as a raw material with zero embedded emissions.
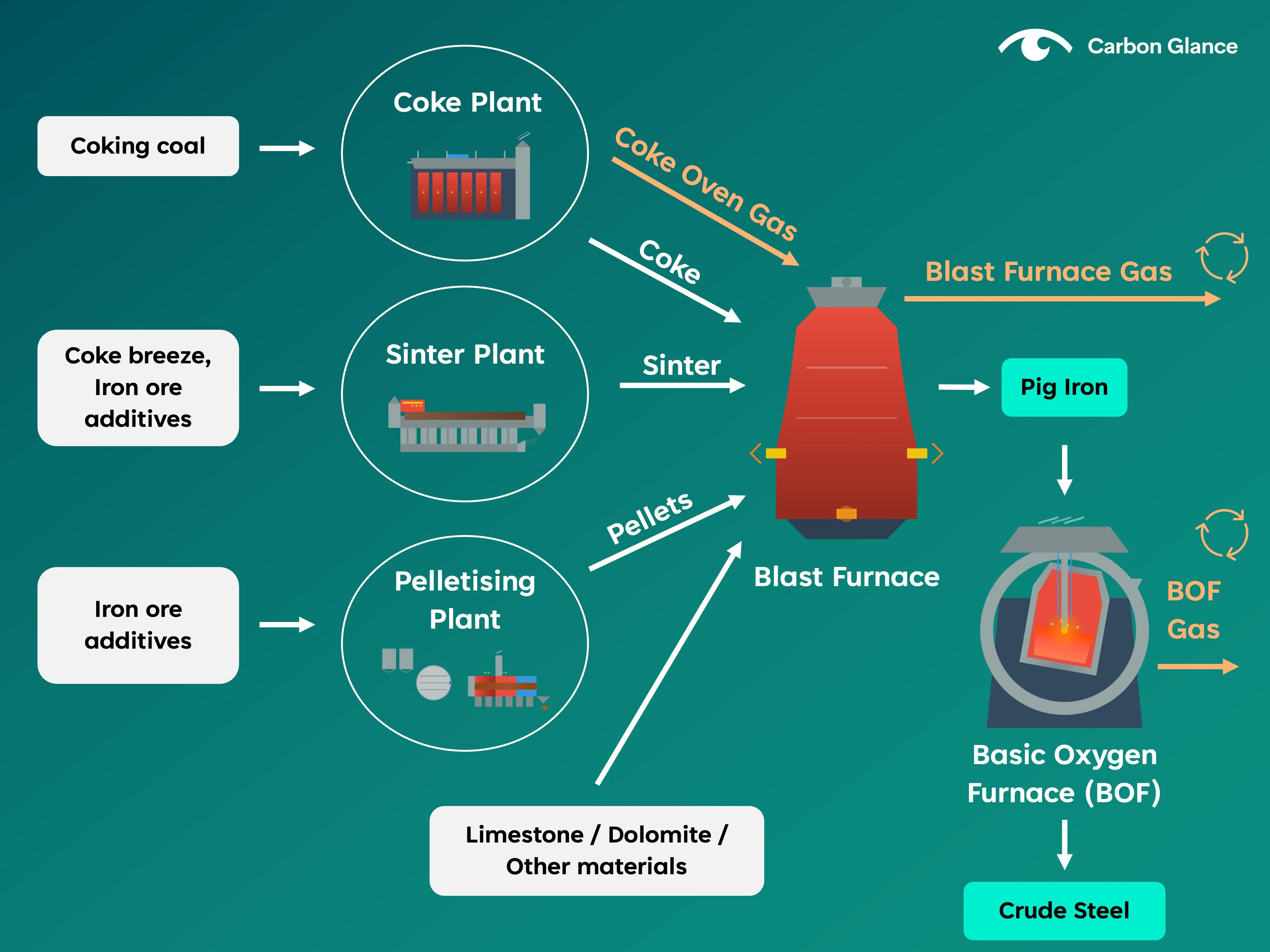
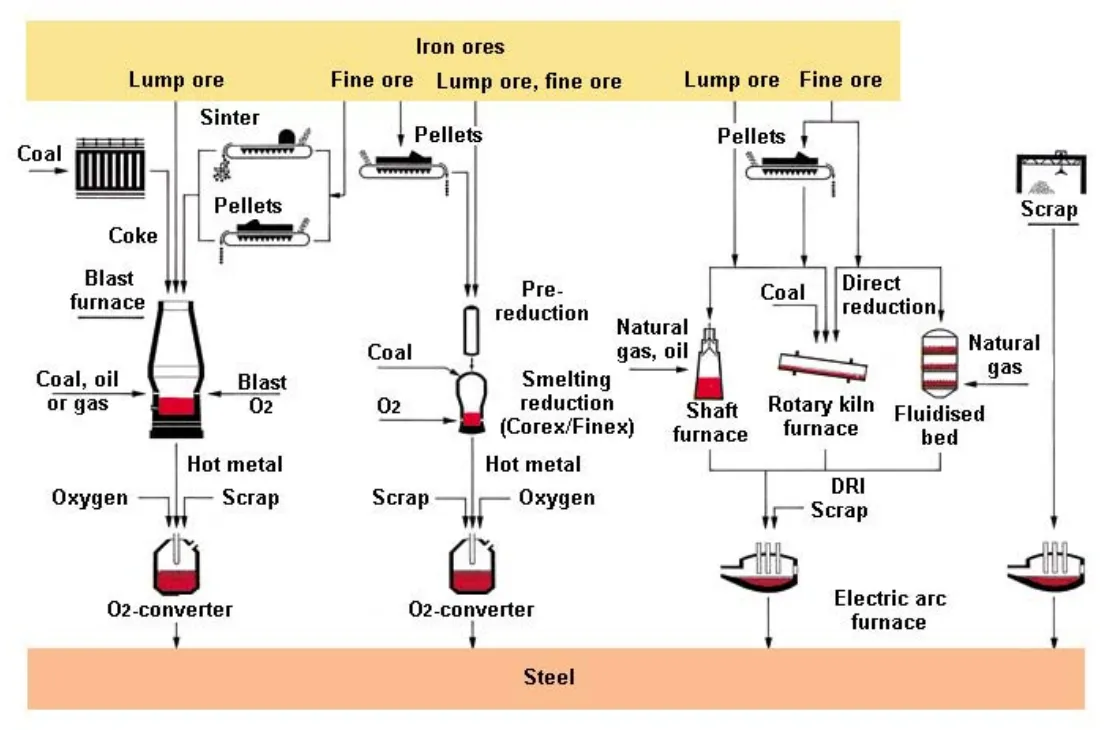
EU CBAM Reminder: The EU CBAM covers all types of iron ore pellet and sinter production.
The sintering process takes place at a sinter plant, where iron ores are aggregated at high temperatures to form sinter. This sinter is an optimal feedstock for the blast furnace. For various reasons, sinter is almost always produced on-site at steelworks.
Iron ore can also be supplied in the form of pellets, which are generally used in the direct reduced iron (DRI) steelmaking method. This process takes place at pelletizing plants, typically located at iron mines or shipping ports, though these plants can also be part of an integrated iron and steel facility.
In general, iron ore products for ironmaking can be classified as follows:
- Lump: Can be fed directly to blast furnaces or direct reduction units.
- Sinter: Produced by sintering and then fed into blast furnaces.
- Pellets: Produced by pelletizing and then fed into blast furnaces or direct reduction units.
In the sintering process, fine-grained, smeltable ores, especially iron ore, are consolidated into compact lumps by heating them nearly to their melting or softening point.
A mixture of iron ore powder, fluxes (such as limestone or dolomite), coke breeze (small-grade oven coke), other additives (such as olivine), recycled sinter from sinter screening, and other recycled substances from downstream iron and steelmaking operations (including, but not limited to, coarse dust and sludge from blast furnace gas cleaning) is mixed in a rotary drum and burned in a sinter machine. The resulting sinter is then crushed, cooled, and screened for dust.
The coke breeze may be produced onsite in coke ovens at integrated iron and steel plants, or it may be purchased from offsite coke producers. Anthracite can be used as a more economical alternative to coke breeze.
Blast furnace gas or coke oven gas produced onsite during integrated iron and steel production may also be used in sinter plants.
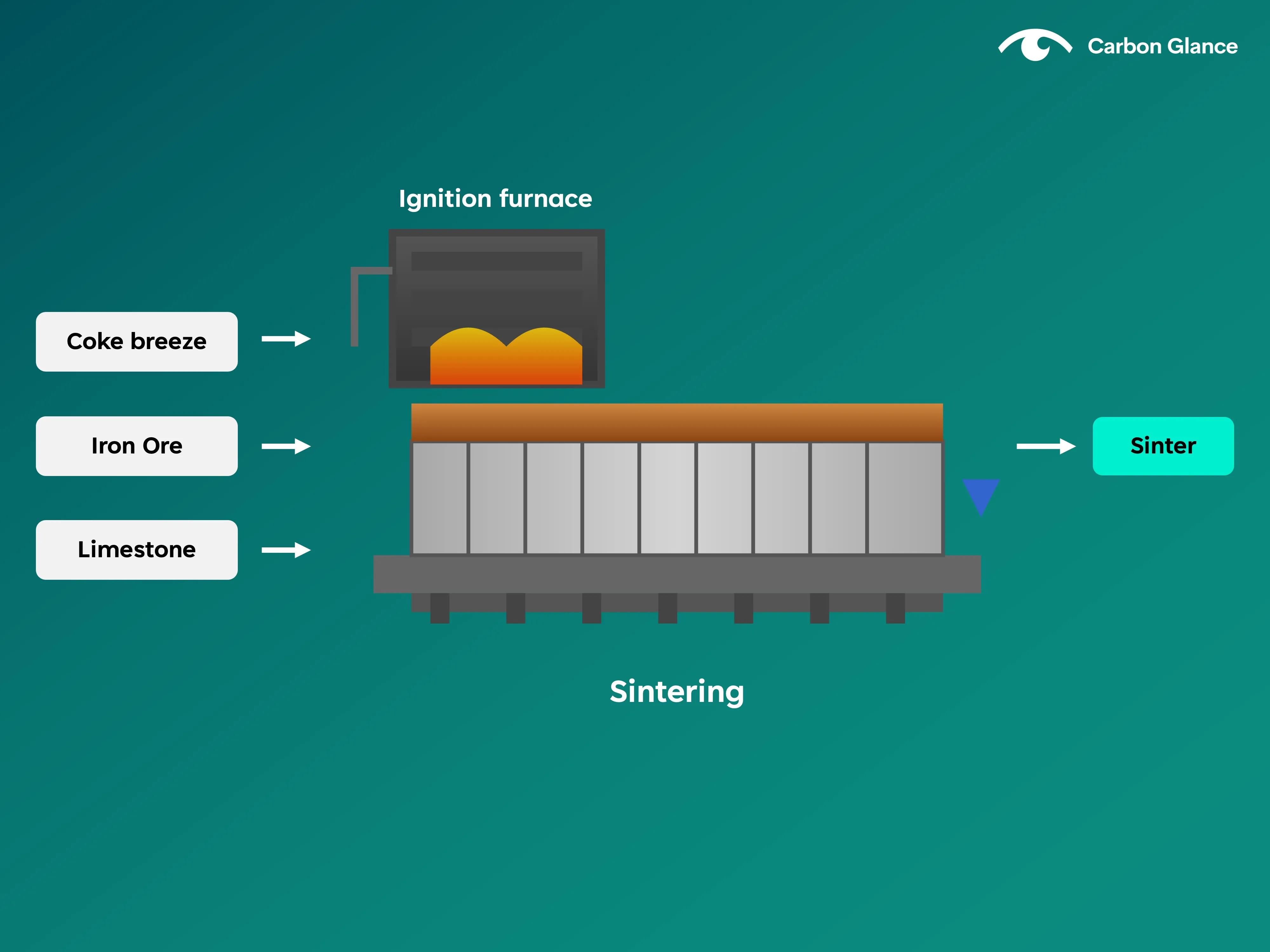
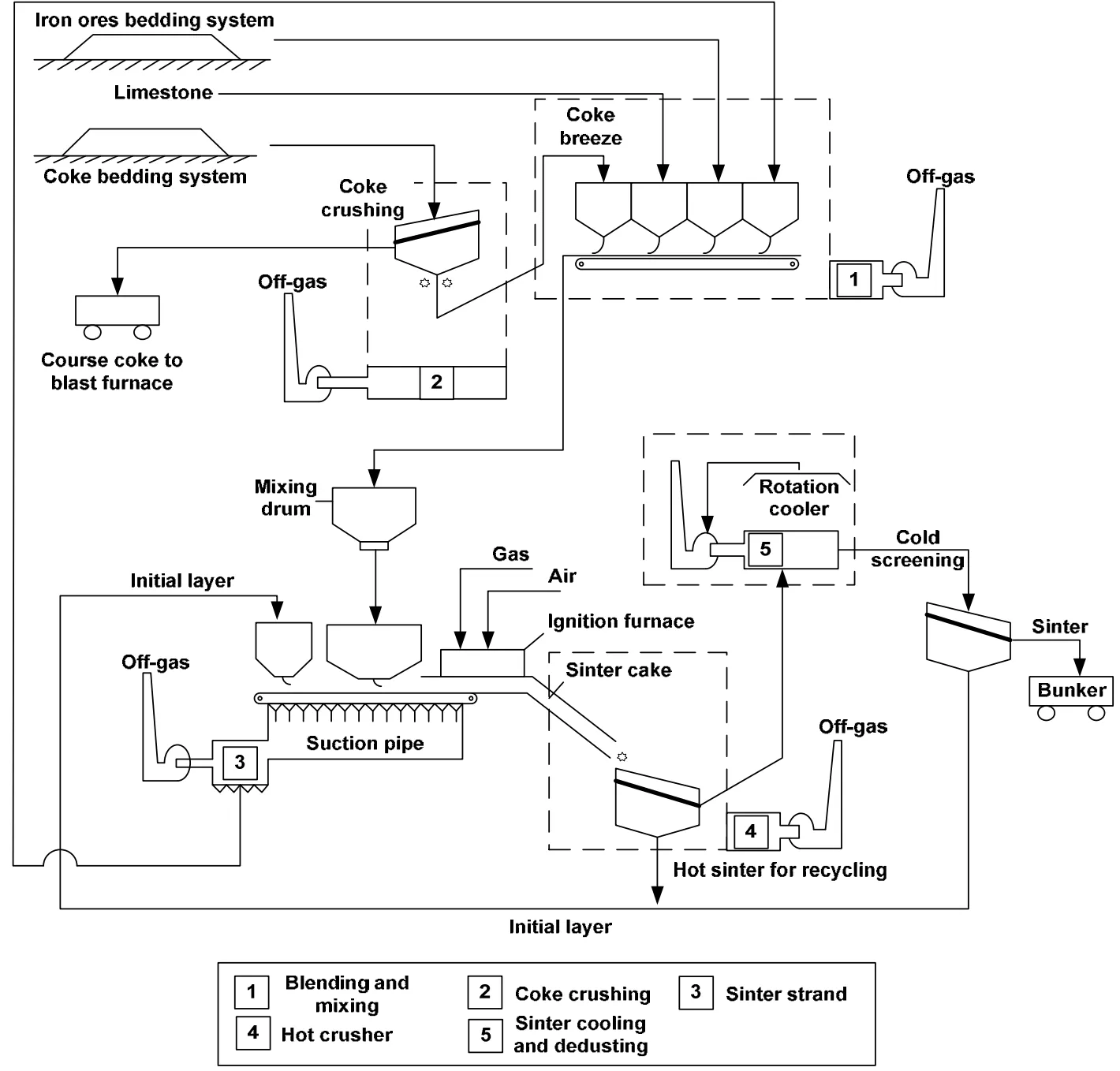
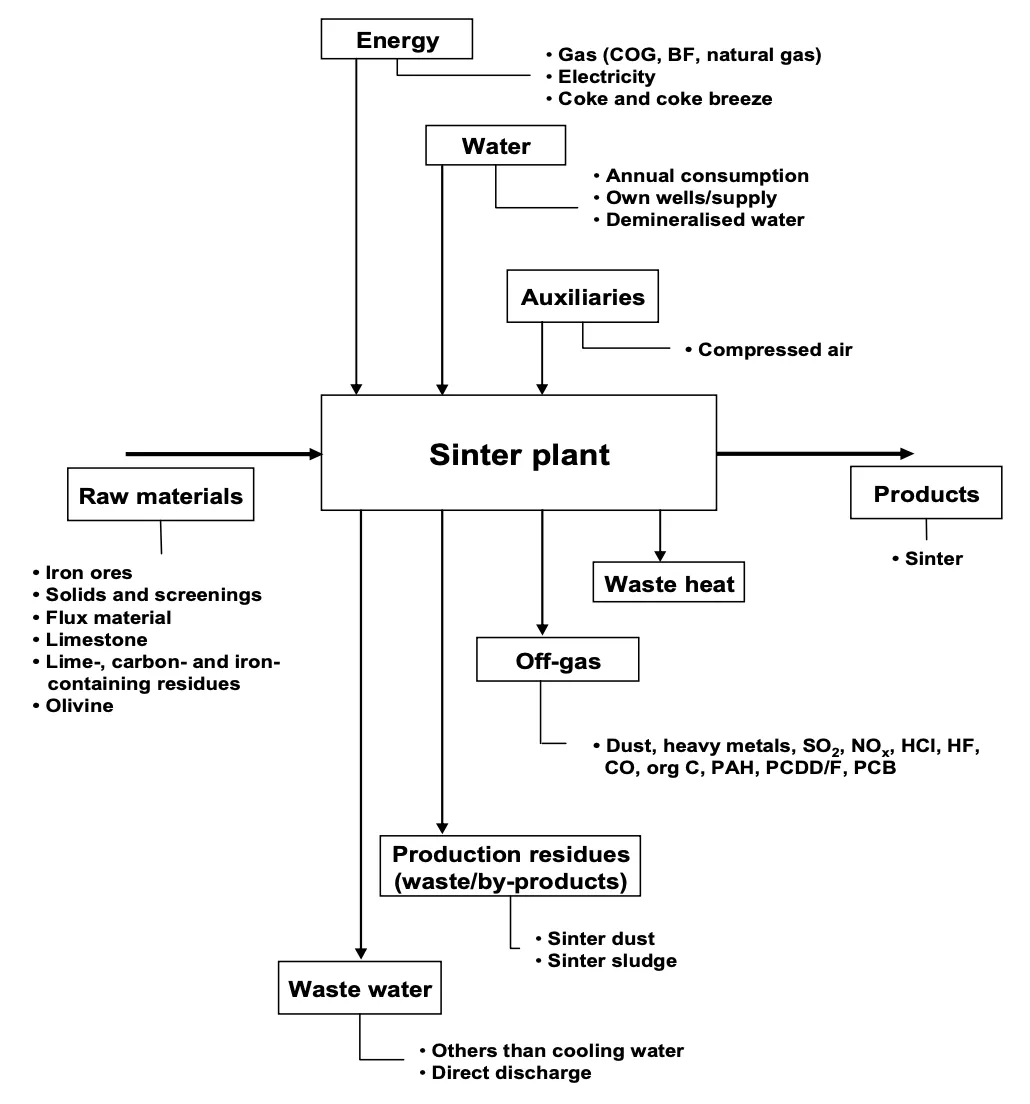
The EU CBAM covers the following production steps for Sintering: |
---|
Raw material handling and pre-treatment; |
Mixing and blending of raw materials; |
Sintering in a furnace, crushing, screening (separation of the pieces to be used in the blast furnace from the pieces to be returned to the sinter process), conveying and cooling; |
Emissions control, in particular waste gas treatment. |
The diagram below shows the example of an installation producing 3,650,000 tonnes of sinter (a standalone sinter plant is depicted to illustrate the main inputs; however, these plants are typically integrated into steel production facilities):
Please note that these values are purely for explanatory and illustrative purposes, as each factory will have its unique design
Inputs
Raw materials:
- Iron ore: 2,968,315 tonnes → no GHG emissions
- Calcium carbonate (limestone): 478,515 tonnes --> 210,546.60 tCO2e (direct emissions)
Intermediate Goods:
- Lime: 37,230 tonnes → no GHG emissions
Fuels:
- Coke breeze: 156,400 tonnes → 449,619.66 tCO2e (direct emissions)
Electricity:
- Grid: 100,000 MWh → 70,000 tCO2e (indirect emissions)
Resulting in the following direct and indirect specific embedded emissions (SEE):
- Direct: 0.1809 tCO2e/tonne
- Indirect: 0.0192 tCO2e/tonne
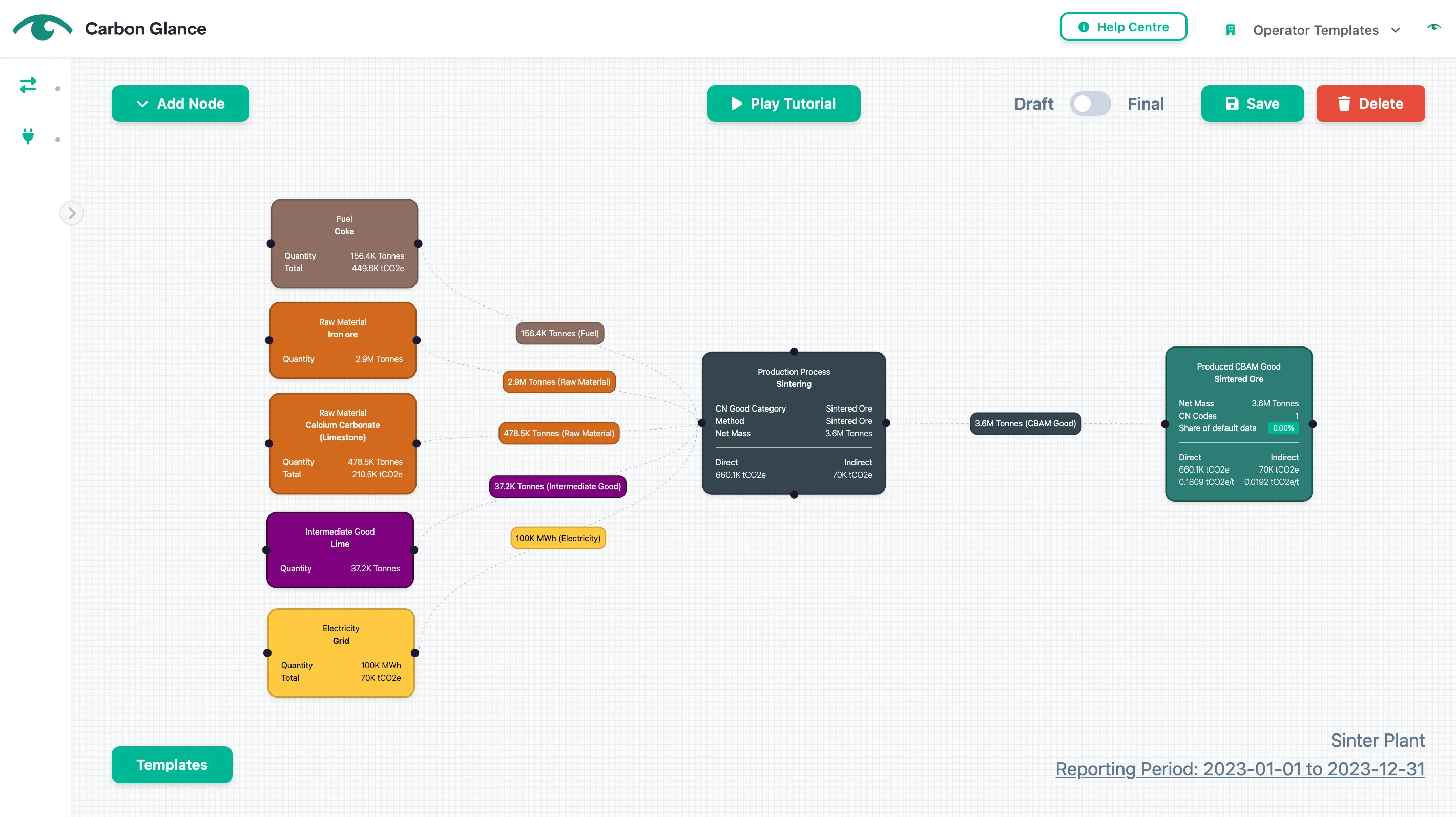
Pellets are formed from iron-containing raw materials, such as fine ore and additives. The pelletisation process involves several stages: grinding and drying or dewatering, wetting and mixing, balling, induration, followed by screening and handling.
Mixing: Finely ground iron ore concentrate is combined with fluxing agents like limestone or dolomite, and binding agents such as bentonite or organic binders, depending on the pellet type. For hematite ores, coke or anthracite coal may be added as an internal fuel to assist in firing the pellets. This mixture is blended in a mixer.
Balling: The blended mixture is fed into balling discs or drums to form spherical green (unfired) pellets.
Induration: These green pellets undergo thermal treatment in an induration furnace, requiring high temperatures. The choice of fuel for this process depends on availability and cost.
Cooling and Handling: After induration, the pellets are cooled to a suitable temperature for transport to a load-out facility.
For pelletisation plants located onsite at an integrated iron and steel facility, coke oven gas may be used as a fuel.
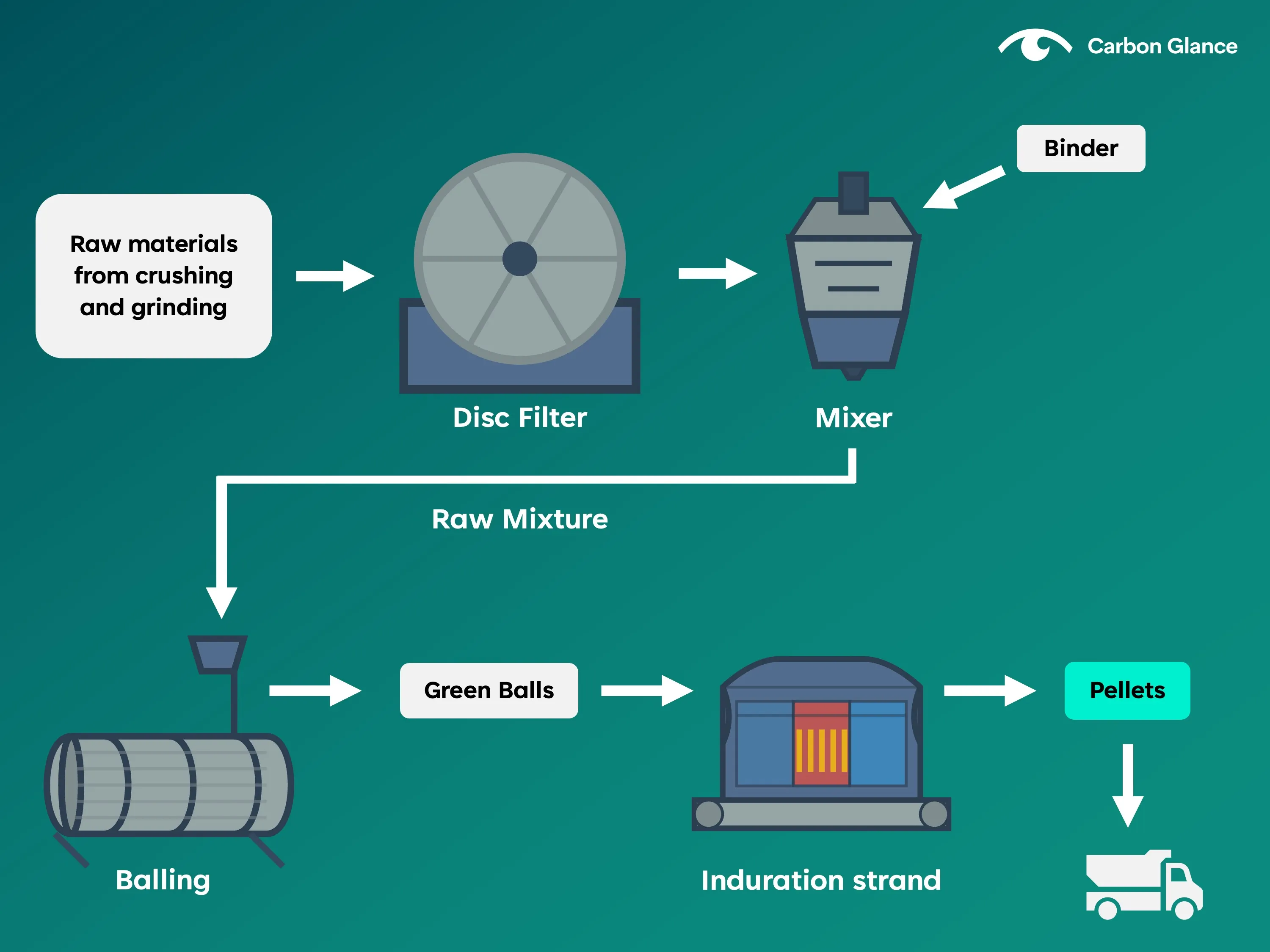
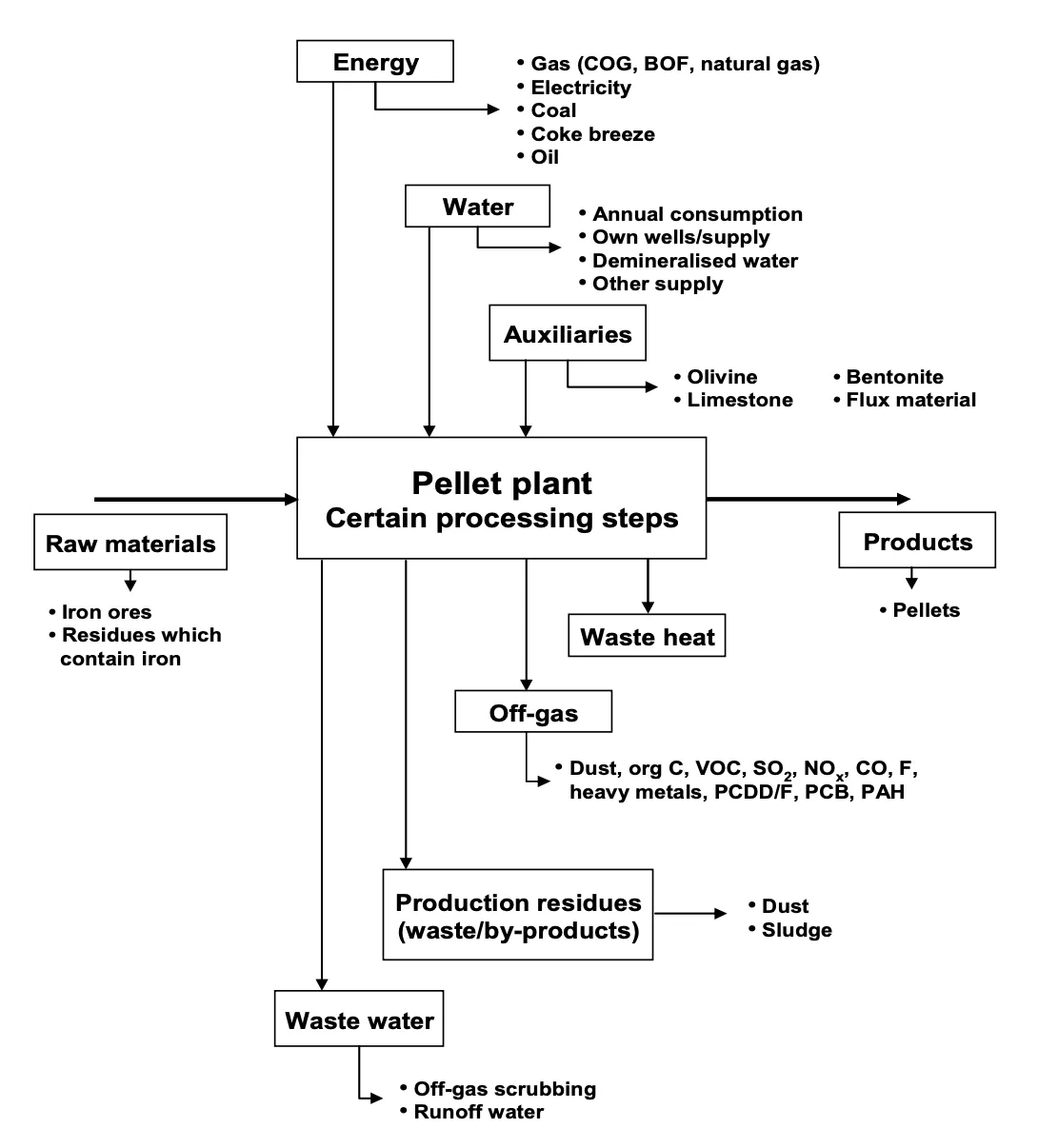
The EU CBAM covers the following production steps for Pelletising: |
---|
Raw material handling and pre-treatment; |
Mixing and blending of raw materials; |
Forming into pellets (balling), thermal treatment (induration), and screening; |
Emissions control, in particular waste gas treatment. |
The diagram below shows the example of an installation producing 3,650,000 tonnes of pellets:
Please note that these values are purely for explanatory and illustrative purposes, as each factory will have its unique design
Inputs
Raw materials:
- Iron ore: 3,467,500 tonnes --> no GHG emissions
- Bentonite: 19,892.5 tonnes --> no GHG emissions
- Dolomite: 24,637.5 tonnes → 11,826 tCO2e
- Calcium carbonate (limestone): 9,125 tonnes --> 4,015 tCO2e
Fuels:
- Coking coal: 28,876 tonnes --> 77,033.08 tCO2e (direct emissions)
- Heavy Fuel Oil (HFO): 10,370 tonnes --> 32,426.58 tCO2e (direct emissions)
- Natural gas: 1,331,510 m3 → 2,868.39 tCO2e (direct emissions)
Electricity:
- Grid: 77,563 MWh → 45,265.77 tCO2e (indirect emissions)
Resulting in the following direct and indirect specific embedded emissions (SEE):
- Direct: 0.0351 tCO2e/tonne
- Indirect: 0.0124 tCO2e/tonne
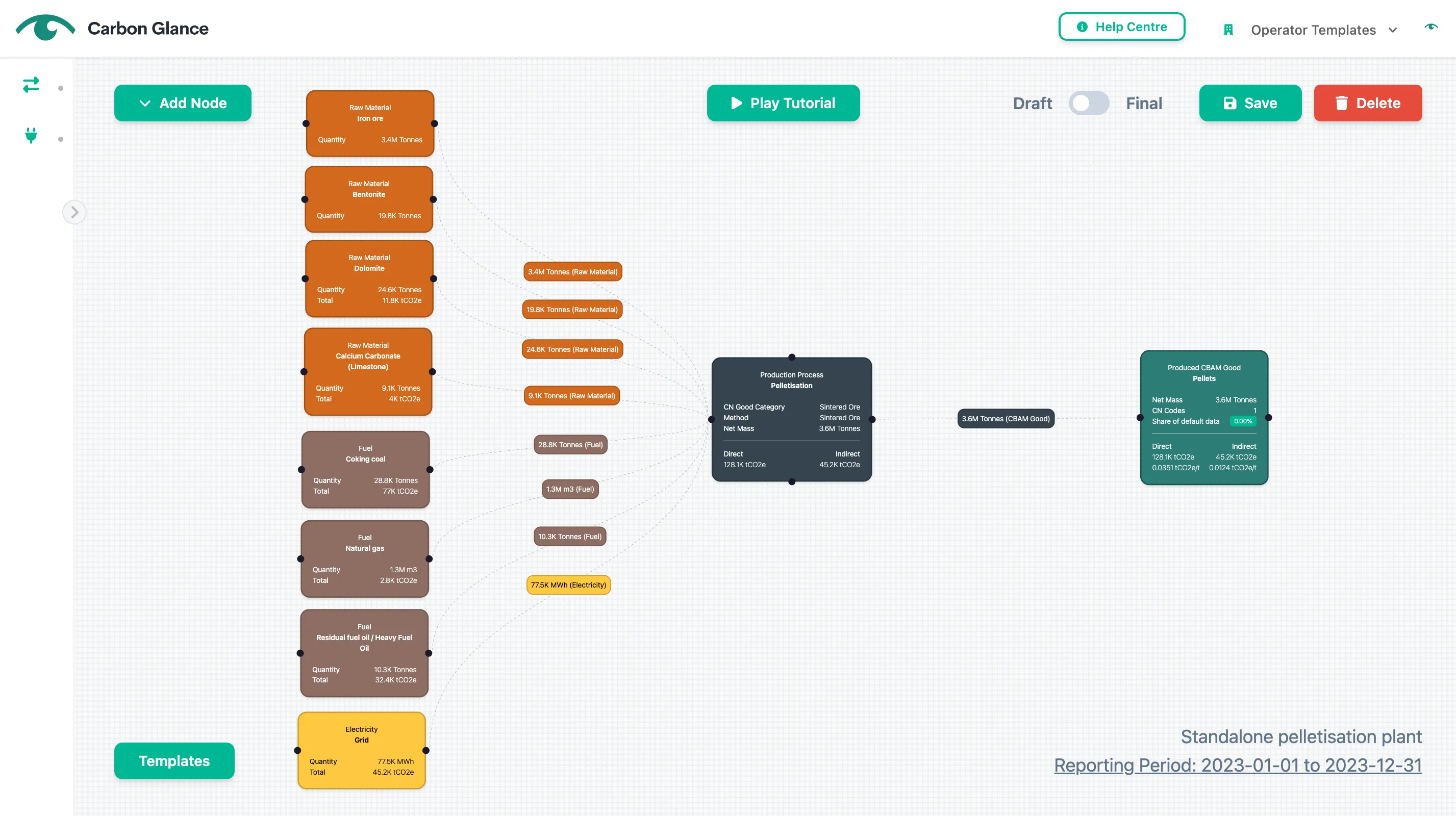
Ferro-alloys are master alloys containing iron and one or more non-ferrous metals as alloying elements. These alloys are primarily used in the production of steel and cast iron to impart specific properties, such as increased strength, hardness, or resistance to corrosion and wear. Ferro-alloys are typically classified into two groups:
- Bulk ferro-alloys: ferro-chrome, ferro-silicon together with silicon metal, ferro-manganese and ferro-nickel
- Special ferro-alloys: ferro-titanium, ferro-vanadium, ferro-tungsten, ferro-niobium, ferro-molybdenum, ferro-boron, alloyed or refined ferro-silicon, silicon metal and ternary/quaternary alloys
1️⃣ EU CBAM Reminder: If you operate a plant producing ferro-alloys, you should use the mass balance method to calculate emissions. Carbon remaining in the product or in slags or wastes (e.g., waste gases) is taken into account using this method.
2️⃣ EU CBAM Reminder: This process covers only the production of the alloys identified under CN codes 7202 1, 7202 4 and 7202 6. Other iron materials with significant alloy content such as spiegeleisen are not covered.
3️⃣ EU CBAM Reminder: Where waste gases or other flue gases are emitted without abatement, CO contained in the waste gas shall be considered as the molar equivalent of CO2 emissions.
Ferro-manganese (FeMn) is a ferro-alloy made by combining iron (Fe) and manganese (Mn). Initially employed as a deoxidising and desulphurising agent, it is primarily used now in steel production to improve the hardness and wear resistance of steel.
Ferro-manganese can be classified as:
- High-carbon ferro-manganese (HC FeMn) --> Max carbon content of 7.5%
- Medium-carbon ferro-manganese (MC FeMn) --> Max carbon content of 2.5%
- Low-carbon ferro-manganese (LC FeMn)--> Max carbon content of 0.75%
The production of ferro-manganese is based on a blend of ores that contain manganese as a primary raw material. Other raw materials that are required for the smelting process are iron ore and fluxing agents such as limestone and dolomite. Coke and low volatile coal are used as a reducing agent and, in the case of HC FeMn production in a blast furnace, also as an energy source.
Electric arc furnaces for the production of ferro-manganese are operated only with self-baking Søderberg electrodes.
The EU CBAM covers the following production steps for ferro-manganese:
- Raw material handling and pre-treatment;
- Electric Arc Furnace (EAF) process - all steps for the EAF process, including charging, melting, primary refining and tapping of the primary furnace;
- Decarburisation and secondary metallurgy - if required to produce ferro-alloys with different carbon contents;
- Casting plant - including casting and cutting, casting ingots pre-heating stands;
- Crushing and granulation;
- Emissions control - for treating releases to ai, water or ground, including dedusting units, post-combustion unit, slag handling.
High-carbon ferro-manganese (HC FeMn)
High-carbon ferro-manganese (HC FeMn) is produced by the carbothermic reduction of lumpy or sintered manganese ore in a three-phase submerged electric arc furnace. The commonly used electric arc furnaces are the closed, semi-closed and open types, which has no or only limited consequences on the furnace itself, but does affect the gas composition, flow rate, recovery and dedusting system used.
The molten metal and the slag can be tapped off continuously or at regular intervals. The metal is cast into moulds lined with crushed ferro-manganese. A casting machine can also be used for casting the liquid metal. The solidified metal is then crushed and screened for the specific needs of the customers. Slag is normally obtained as rich slag (with about 30 % manganese) and is used further for the production of silico-manganese.
There are some facilities where the CO-rich off-gas is used to produce electricity. The CO-rich gas can also be utilised for other industrial uses, for instance as a raw material for chemical synthesis.
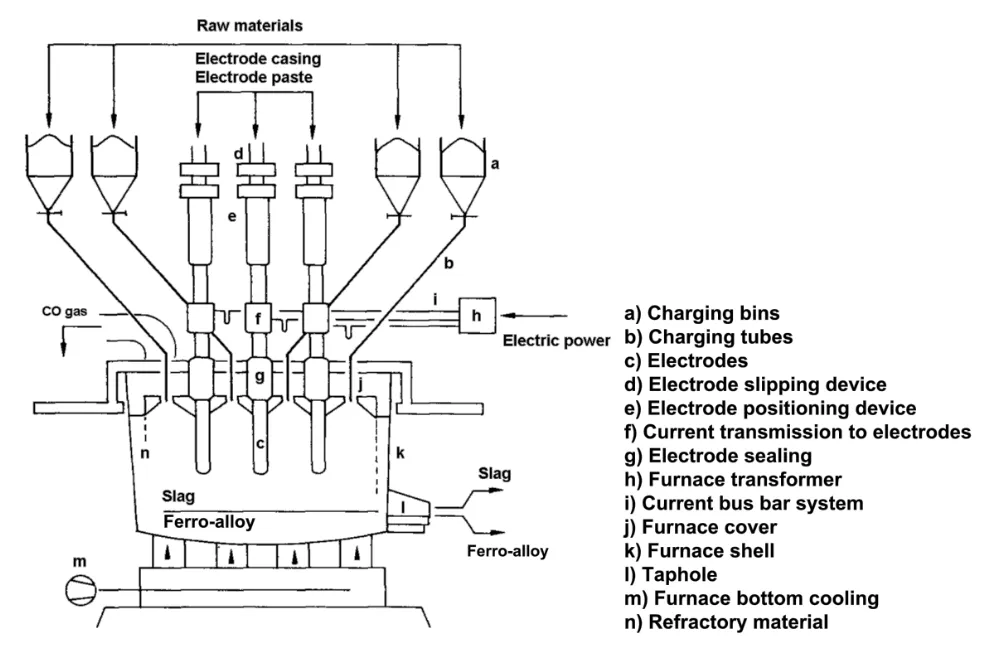
The diagram below shows the example of an installation producing 180,000 tonnes of high-carbon ferro-manganese (HC FeMn):
Please note that these values are purely for explanatory and illustrative purposes, as each factory will have its unique design
Inputs
Raw materials:
- Manganese ore: 450k tonnes --> no GHG emissions
- Limestone: 90k tonnes --> 39,600 tCO2e
Fuels:
- Coking coal: 130k --> 346,803.60 tCO2e (direct emissions)
Intermediate goods:
- Electrodes: 4.2k tonnes --> 12,600 tCO2e
Electricity:
- Grid: 540k MWh → 315,144 tCO2e (indirect emissions)
Resulting in the following direct and indirect specific embedded emissions (SEE):
- Direct: 2.2167 tCO2e/tonne
- Indirect: 1.7508 tCO2e/tonne
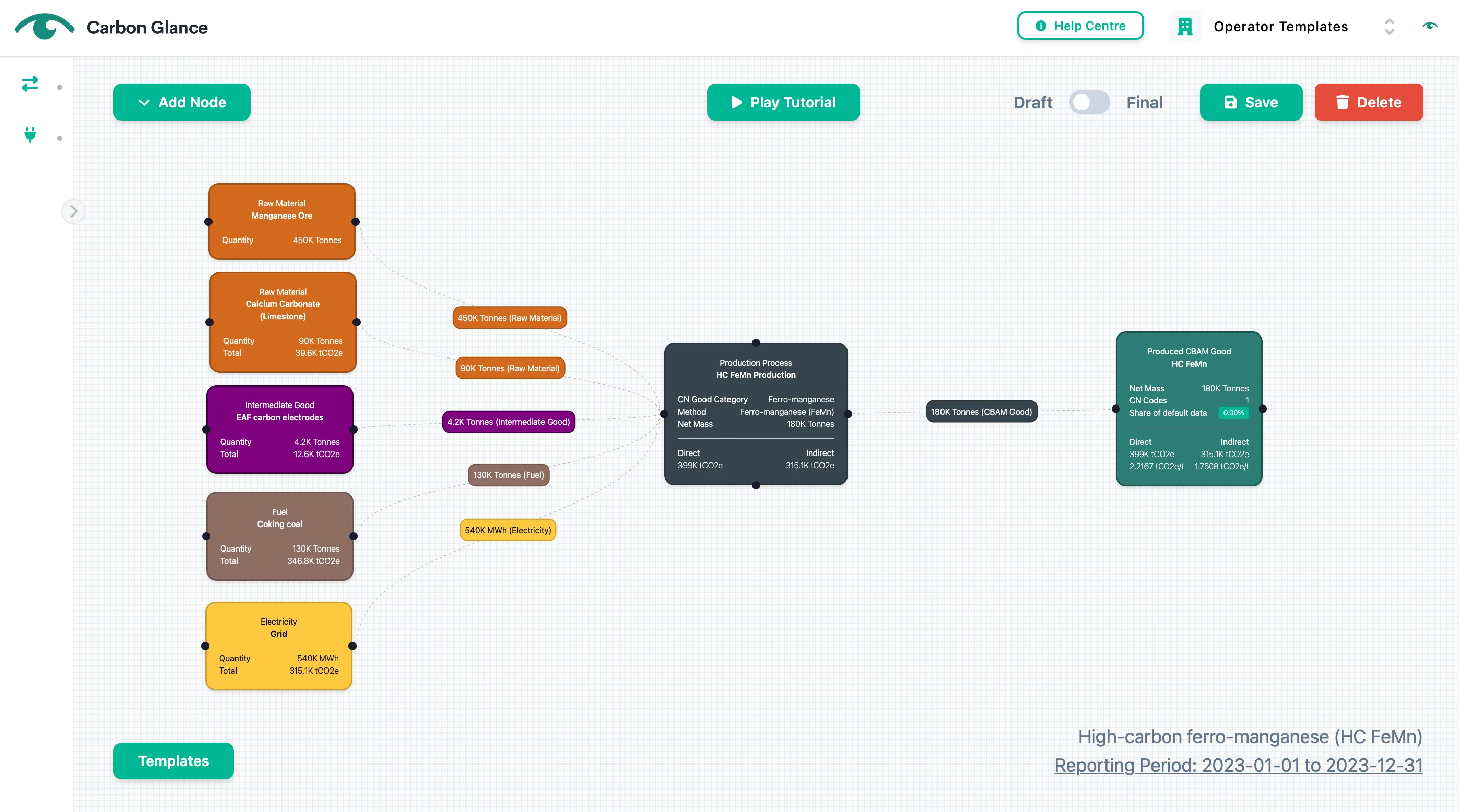
Medium-carbon ferro-manganese (MC FeMn)
For the production of medium-carbon ferro-manganese (MC FeMn) two different routes can be used:
- The decarburisation of HC FeMn in an oxygen-blown converter: in this process, oxygen is blown into the molten metal. The oxygen blown into the melt oxidises part of the manganese and increases the bath temperature. With increasing temperatures, the carbon present in the HC FeMn also tends to be oxidised which again raises the temperature.
- The silicothermic reduction of manganese ore (see below).
Low-carbon ferro-manganese (LC FeMn)
Low-carbon ferro-manganese (LC FeMn), which usually contains less than 0.75 % carbon, is traditionally produced by a silicothermic process route. This process needs manganese ore and lime or a high-grade slag and silico-manganese as input materials. The production of silico-manganese is based on manganese ore or sinter and quartz as raw material.
The production of LC FeMn takes place in a three-phase electric electric arc furnace. The slag obtained is used for silico-manganese production as a raw material or sold for other applications in the metallurgical industry.
Ferro-chromium or ferro-chrome (FeCr) is a ferro-alloy composed of iron (Fe) and chromium (Cr). Ferro-chrome, along with nickel (ferro-nickel), is the major alloying element in the production of stainless steel. In fact, stainless steel is mainly produced from Cr-containing scrap metal and FeCr.
The main raw material to produce ferro-chrome is chromite ore, which is a mineral that contains iron and chromium oxides. Chromite ore is used in the form of hard or upgraded lump varieties, friable lumps or fine concentrate. To convert ore fines and concentrates to furnaceable material, they first need to be agglomerated by briquetting, pelletising and sintering, or sintering alone.
The chromium content of ferro-chrome normally varies from 45% to 75% and it also contain various amounts of iron, carbon and other alloying elements. The use of ferro-chrome depends on the carbon content. Ferro-chrome can be classified as:
- High-carbon ferro-chrome (HC FeCr) --> 2% - 12% carbon
- Medium-carbon ferro-chrome (MC FeCr) --> 0.5% - 4% carbon
- Low-carbon ferro-chrome (LC FeCr) with 0.01% - 0.5% carbon
Depending on the different production routes and the desired carbon content of the ferro-chrome, carbon or silicon is used as a reducing agent. In addition to reductants, fluxes are also used during smelting, e.g., dolomite, quartzite, olivine, bauxite, limestone, and calcite, depending on the operation conditions, furnace type, smelting regime, and the chemical composition of the chromite.
The EU CBAM covers the following production steps for ferro-chromium:
- Raw material handling and pre-treatment - Agglomeration of the ore fines and concentrates by briquetting, pelletising and sintering
- Electric Arc Furnace (EAF) process - all steps for the EAF process, including charging, melting, primary refining and tapping of the primary furnace
- Decarburisation and secondary metallurgy - if required to produce ferro-alloys with different carbon contents
- Casting plant - including casting and cutting, casting ingots pre-heating stands
- Crushing and granulation
- Emissions control - for treating releases to ai, water or ground, including dedusting units, post-combustion unit, slag handling
High-carbon ferro-chrome (HC FeCr)
High-carbon ferro-chrome (2% - 12% carbon) is made by combining chromium ore with carbon in three-phase submerged electric arc furnaces (closed, semi-closed, or open types) operating continuously. These furnaces use Søderberg electrodes. For the production of high-carbon ferro-chrome (HC FeCr), carbon is added to the process as a reducing agent in the form of metallurgical coke (coking coal), coal, or charcoal. Metallurgical coke is the most common reducing agent.
In the reduction process, metal oxides react with coke to form metal carbides, generating substantial carbon monoxide (CO) gas. While open furnaces burn this gas at the surface, closed furnaces reduce off-gas volume by 50-75 times and semi-closed by 10-20 times, allowing the captured gas to be repurposed for raw material preheating.
The process outputs ferro-chrome and slag are tapped through the same tapholes. The ferro-chrome is either bed/layer cast and processed into commercial grades through crushing and screening. If possible, the molten ferro-chrome can also be transferred directly to an adjacent stainless steelmaking plant.
The slag can either be granulated with a high-pressure water jet or cast outdoors in layers, cooled, crushed and screened. Both the granulated and the lump slag are reused as building and road construction material.
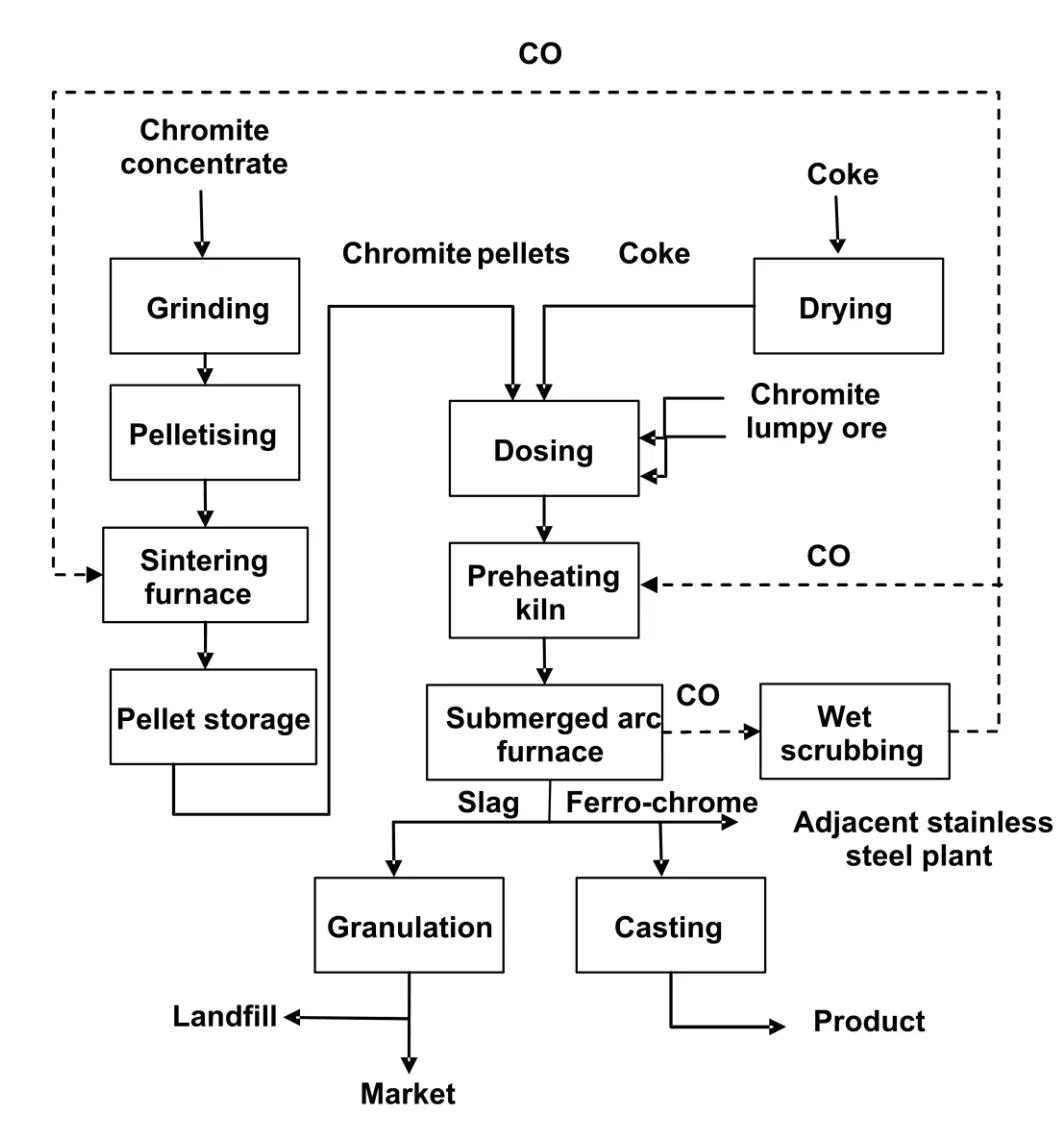
The diagram below shows the example of an installation producing 420,000 tonnes of high-carbon ferro-chrome (HC FeCr):
Please note that these values are purely for explanatory and illustrative purposes, as each factory will have its unique design
Inputs
Raw materials:
- Chromite pellets: 1.1M tonnes --> no GHG emissions
- Calcium carbonate (limestone): 100k tonnes --> 44,000 tCO2e
Fuels:
- Coking coal: 350k --> 933,702 tCO2e (direct emissions) --> coke is used primarily as a reducing agent. While electricity provides the main heat input through the electrodes, the coke also contributes to the thermal energy through its reaction with metal oxides (exothermic reactions).
Intermediate goods:
- Electrodes: 4.2k tonnes --> 12,600 tCO2e
Electricity:
- Grid: 1,470,000 MWh → 857,892 tCO2e (indirect emissions)
Resulting in the following direct and indirect specific embedded emissions (SEE):
- Direct: 2.2231 tCO2e/tonne
- Indirect: 2.5011 tCO2e/tonne
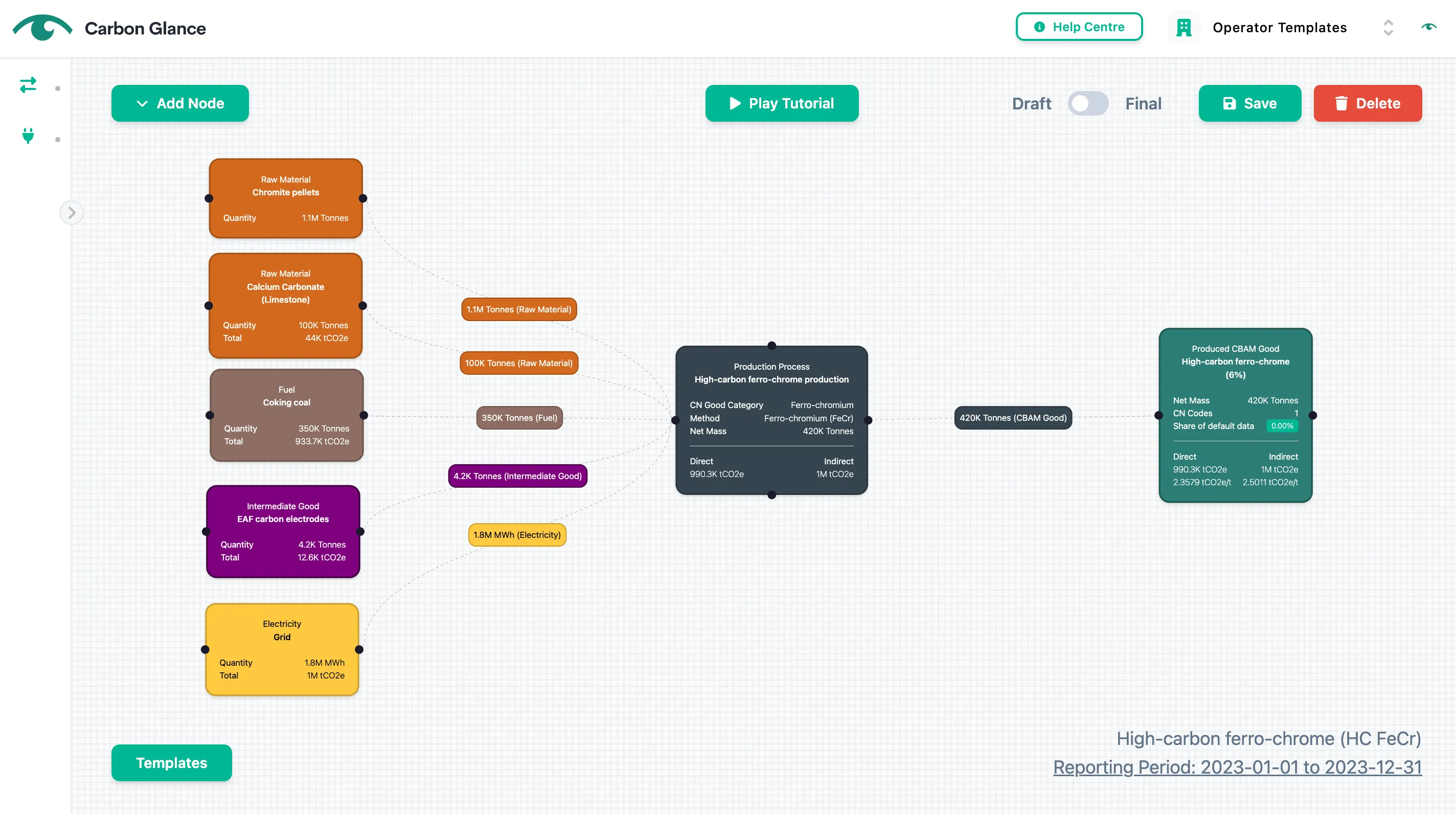
Medium-carbon ferro-chrome (MC FeCr)
Medium-carbon ferro-chrome (0.5% - 4% carbon) can be produced by:
- the silicothermic reduction of chromite ore and concentrates; or
- the decarburisation of HC FeCr in an oxygen-blown converter.
Low-carbon ferro-chrome (LC FeCr)
Low-carbon ferro-chrome (0.01-0.5% carbon) production cannot use a carbothermic process because using carbon as a reducing agent would result in high carbon content in the final product. Instead, metals like aluminum or silicon (typically as ferro-silicon) are used as reducing agents in what's known as metallothermic reduction. These can be the Simplex, Duplex or Perrin processes.
In the Duplex and Perrin processes, high-carbon ferro-chrome is used as a starting material/input to produce low-carbon ferro-chrome.
Simplex: In this process, HC FeCr is crushed in a ball mill in order to get a ferro-alloy powder. After briquetting the HC FeCr powder together with chromic oxide (Cr2O3) and iron oxide (Fe2O3), the mixture can be decarburised by annealing at about 1,350°C in a vacuum furnace.
Duplex: In this process, LC FeCr is produced by the silicothermic reduction of a lime-chromite slag. The slag smelting process takes place in a tiltable alternating current (AC) arc furnace with Søderberg electrodes. The furnace is operated at temperatures above 1,500°C. The liquid melt is tapped at regular intervals into a first reaction ladle. When SiCr, ore and lime are added to the laddle, the reacting mixture is converted into LC FeCr and an intermediate slag.
Perrin: This process is similar to the Duplex one, but uses two arc furnaces.
The diagram below shows the example of an installation producing 60,000 tonnes of low-carbon ferro-chrome (LC FeCr):
Please note that these values are purely for explanatory and illustrative purposes, as each factory will have its unique design
Inputs
CBAM Good (Relevant Precursor):
- External Precursors:
- High-carbon ferro-chrome (HC FeCr): 65k tonnes
- Direct embedded emissions: 134,550 tCO2e
- Indirect embedded emissions: 165,100 tCO₂e
Intermediate goods:
- Electrodes: 600 tonnes --> 1,800 tCO2e
- Ferro-silicon --> 12k tonnes --> no GHG emissions
- Lime --> 8k tonnes --> no GHG emissions
Electricity:
- Grid: 70,000 MWh → 40,852 tCO2e (indirect emissions)
Resulting in the following direct and indirect specific embedded emissions (SEE):
- Direct: 0.0300 tCO2e/tonne
- Indirect: 0.6809 tCO2e/tonne
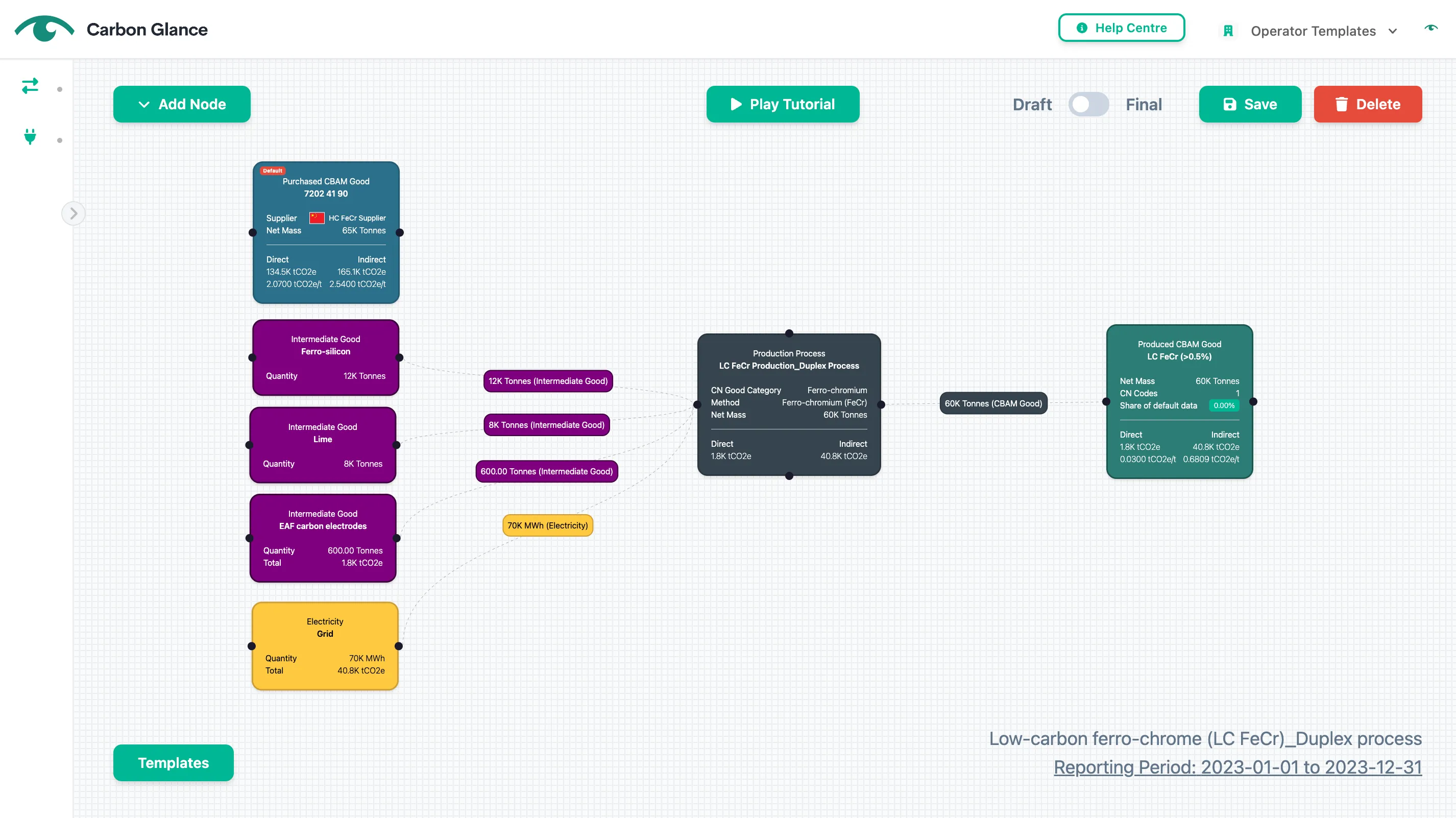
Ferro-nickel (FeNi) is a ferro-alloy composed primarily of iron (Fe) and nickel (Ni). Together with ferro-chrome, ferro-nickel is the major alloying agent in the production of stainless steel due to its ability to impart corrosion resistance, ductility, and heat resistance.
Laterite ore is the main raw material. Besides this raw material, coke and/or coal are also needed in ferro-nickel production. FeNi can also be produced from secondary raw materials, such as spent catalysts and sludge from the galvanising industry.
EU CBAM Reminder:
- If Nickel Content ≥ 10%: Nickel pig iron (NPI) is treated as a ferro-nickel product. This reflects its use as a nickel source in stainless steel manufacturing.
- If Nickel Content < 10%: The product falls under the category of pig iron, specifically that produced via the blast furnace route.
The EU CBAM covers the following production steps for ferro-nickel:
- Raw material handling and pre-treatment (e.g., drying of raw materials)
- Electric Arc Furnace (EAF) process - all steps for the EAF process, including charging, melting, primary refining and tapping of the primary furnace
- Casting plant - including casting and cutting, casting ingots pre-heating stands
- Crushing and granulation
- Emissions control - for treating releases to ai, water or ground, including dedusting units, post-combustion unit, slag handling
Production of ferro-nickel from primary raw materials
Ferro-nickel production from primary raw materials uses the rotary kiln electric furnace process. Due to high moisture content in raw materials, the process begins with drying in a directly fired rotary dryer.
The material is then homogenized, mixing ores with coal, coke, and recycled pelletized dust. This mixture enters a rotary kiln for calcination (preliminary reduction process), which dehydrates the ore and pre-reduces nickel and iron oxide. The resulting feed contains metallic nickel and pre-reduced iron oxides.
The hot calcine is transferred to an electric arc furnace, (also named submerged arc furnace). In the open bath semi-closed electric arc furnace, final smelting occurs using carbon electrodes and reducing agents such as coke and coal.
Ferro-nickel produced by the conventional process needs further refining to remove impurities like sulphur, silicon and phosphorus.
Finally, the purified ferro-nickel is cast into ingots or granulated under water.
The diagram below shows the example of an installation producing 200,000 tonnes of ferro-nickel (FeNi):
Please note that these values are purely for explanatory and illustrative purposes, as each factory will have its unique design
Inputs
Raw materials:
- Laterite ore: 1.2M tonnes --> no GHG emissions
Fuels:
- Coking coal: 180k tonnes --> 480,189.60 tCO2e
Intermediate goods:
- Recycled Pelletized Dust: 25k tonnes --> no GHG emissions
Electricity:
- Grid: 1,320,000 MWh → 770,352 tCO2e (indirect emissions)
Resulting in the following direct and indirect specific embedded emissions (SEE):
- Direct: 2.4009 tCO2e/tonne
- Indirect: 3.8518 tCO2e/tonne
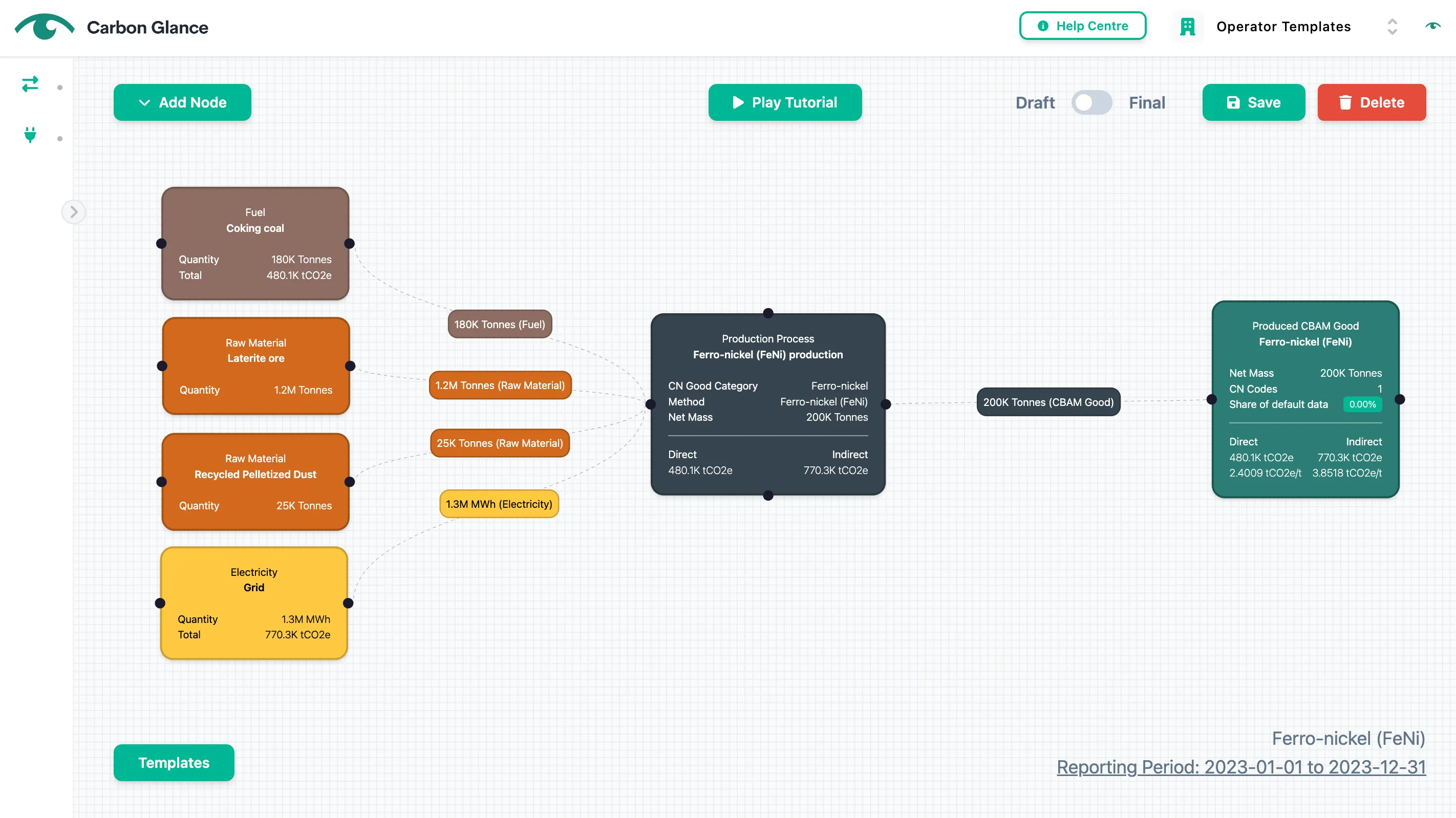
Production of ferro-nickel from secondary raw materials
Ferro-nickel can also be produced from residues that contain nickel. These residues, mostly spent catalysts from grease production, are burnt in a rotary kiln in order to concentrate the nickel content as nickel oxide in the flue-dust.
The production of FeNi then takes place in a submerged electric arc furnace. The molten alloy is tapped, granulated in water and packed in drums or big bags for supply.
The iron-making process consists of using carbon to convert the iron ore (iron oxide) to iron.
Pig Iron (also called "hot metal") can be produced either via the conventional Blast Furnace (BF) route or through the smelting reduction process (COREX and FINEX routes).
1️⃣ EU CBAM Reminder: If you operate a facility that produces pig iron, you should use the mass balance method to calculate your emissions. Carbon remaining in the product or in slags or wastes (e.g., waste gases) is taken into account using this method.
2️⃣ EU CBAM Reminder: Under the EU CBAM, coke is treated as a raw material with zero embedded emissions.
3️⃣ EU CBAM Reminder:
- If Nickel Content ≥ 10%: Nickel pig iron (NPI) is treated as a ferro-nickel product. This reflects its use as a nickel source in stainless steel manufacturing.
- If Nickel Content < 10%: The product falls under the category of pig iron, specifically that produced via the blast furnace route.
The blast furnace is a large industrial furnace that operates at high temperatures to reduce iron oxide into molten iron. This industrial route still produces most of the world's steel. In this process, the materials are continuously charged from the top of the furnace. A hot blast of air is blown into the lower section of the furnace through a series of pipes called tuyeres. The preheated air (blast air) facilitates the chemical reactions needed to produce pig iron. The main reducing agents in a blast furnace are coke and pulverised coal forming carbon monoxide (CO) and hydrogen which reduce the iron oxides. They also partly act as a fuel. Charcoal made from wood may also be used.
The main input materials used in the blast furnace are:
- Sintered ore: Iron ore for the blast furnace is usually supplied in the form of sinter, but lump ore and pellets may be used as well.
- Coking coal (metallurgical coke): Coke is produced from coal by dry distillation in coke ovens at the coke plant. In some countries, coke plants are not integrated with iron and steel production but are located offsite. Since coke is not a CBAM good, it is treated as a raw material with zero embedded emissions. Coke reacts with oxygen in the hot air blast to form carbon dioxide (CO2), releasing heat. The CO2 then reacts with more coke to form carbon monoxide, which ascends through the furnace, reacting with iron ore (iron oxide) and reducing it to iron.
- Limestone/Lime: Limestone is used as a flux to remove impurities such as silica from the iron ore. The limestone decomposes at high temperatures to form lime (CaO) and carbon dioxide. The lime then reacts with silica (SiO2) and other impurities in the iron ore to form slag, which has a lower density than the molten iron and therefore floats.
- Ferro-alloys: If applicable, ferro-alloys are added to meet the desired properties of the iron.
- Additional reducing agents/fuels: In many cases, additional reducing agents/fuels are supplied by the injection of oil, coal tar, natural gas and (in a few cases) plastics, fats, and various gases (e.g., coke oven gas).
- Other materials: This could include plastic, recovered oils fats, magnesite, emulsions, etc.
The main output materials obtained are:
- Molten pig iron: The molten pig iron is tapped from the bottom of the furnace and collected in ladles.
- Slag: The slag is a complex mix of silicates. Due to its lower density, the slag floats on top of the molten iron. The slag is tapped off and removed, with some carbon remaining in it. This is one of the reasons the mass balance method should be used. The slag from the blast furnace is granulated, pelletised, or tapped into slag pits. The resulting materials are usually sold to cement manufacturing companies or used in road construction, among others.
- Blast furnace gas (also referred to as waste gas): Blast furnace gas is produced during the combustion of coke and is the gas with the lowest calorific value and flame temperature. Hence, it is commonly used in low-temperature processes such as the blast furnace itself (hot blast stoves) and the coke oven plant. The stoves of the blast furnace can be heated exclusively on blast furnace (BF) gas. Any remaining BF gas can be used in the power plant to produce electrical power and process steam. Basic oxygen furnace (BOF) gas can be used as an enrichment gas to improve the calorific value of the BF gas.
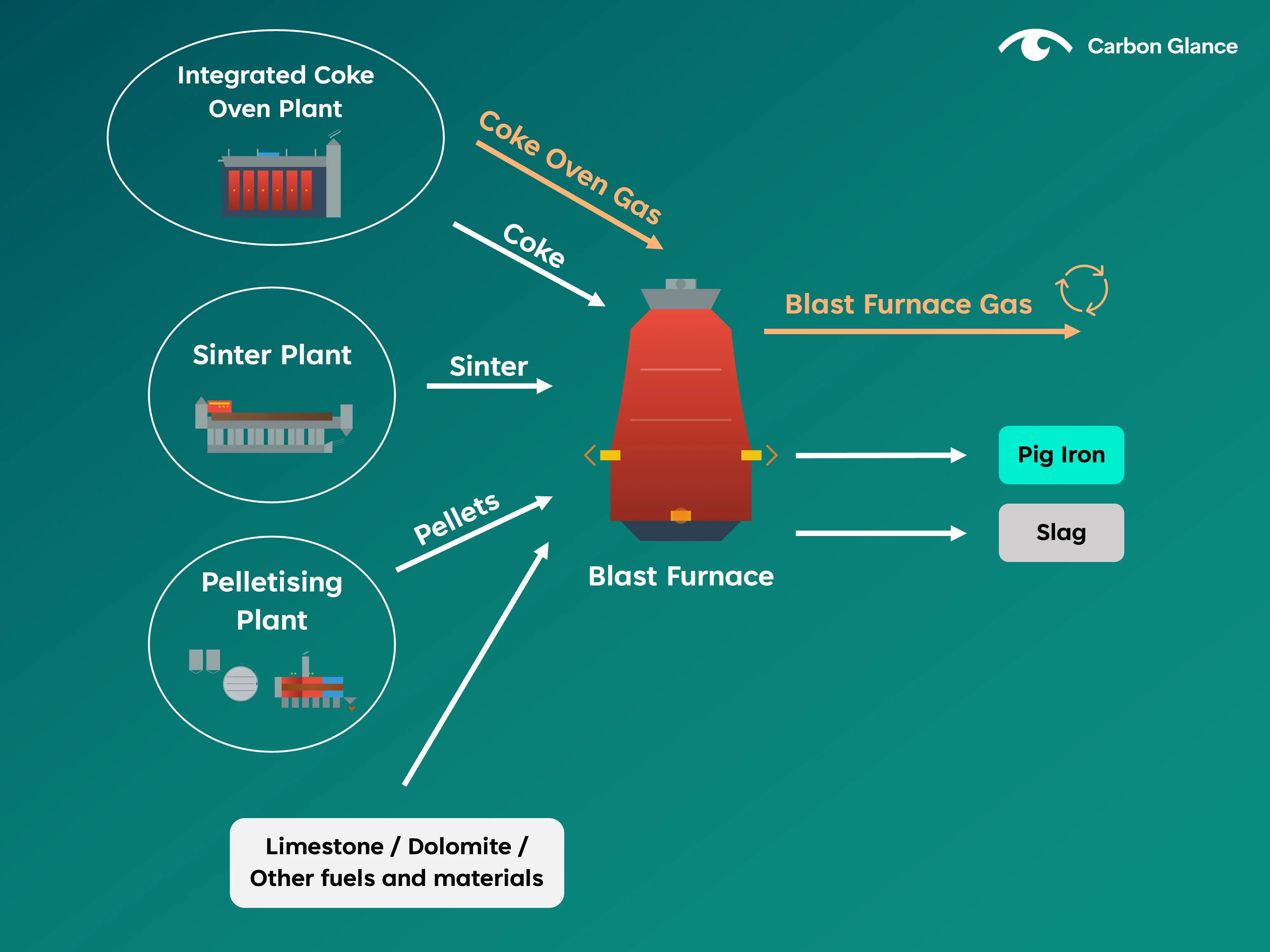
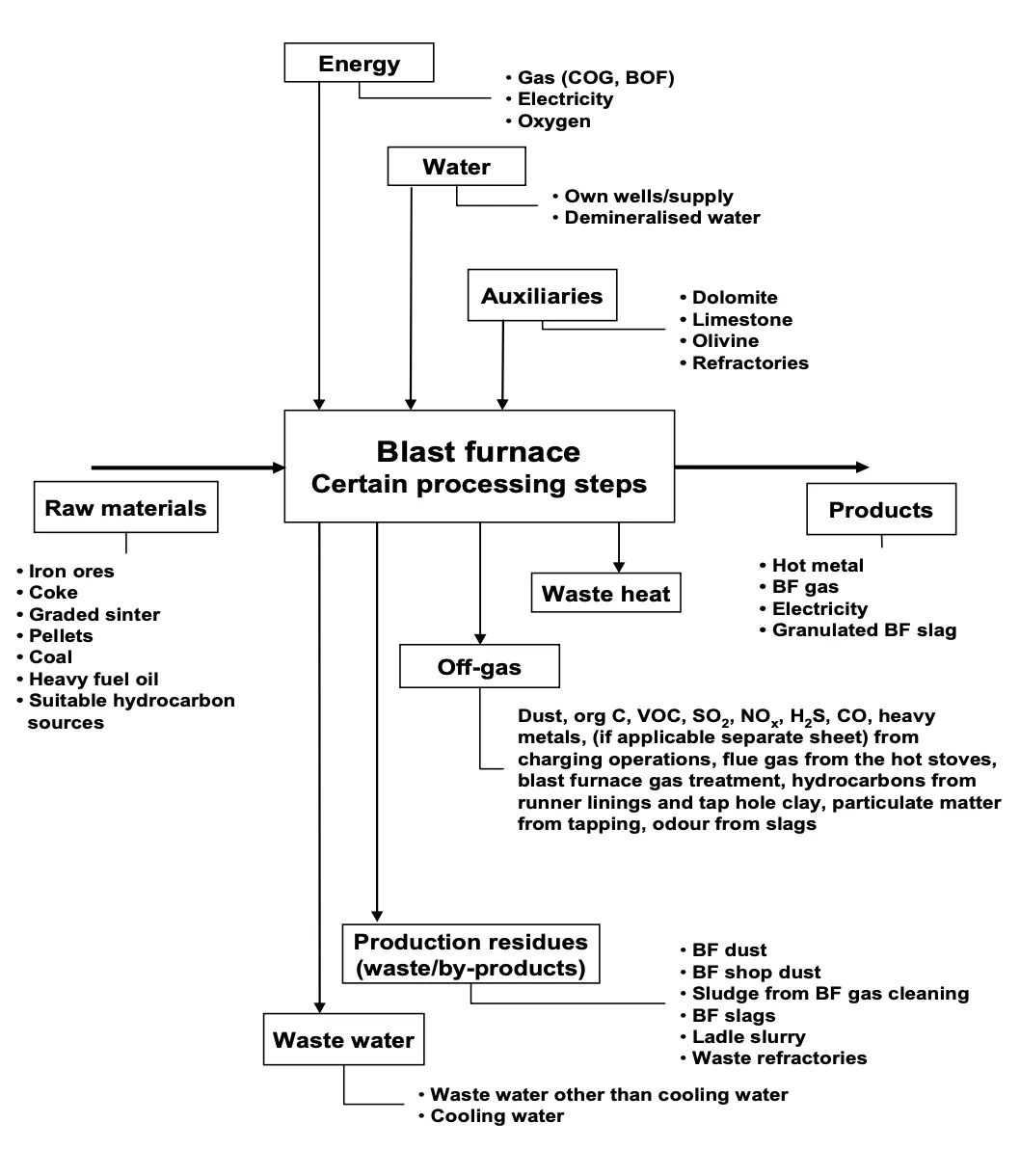
The EU CBAM covers the following production steps for pig iron:
- Raw material handling and pre-treatment;
- Fuel storage and preparation – e.g. coal drying and preparation for pulverized coal injection (PCI) and vessels preheating stands;
- Hot metal production – all steps for the blast furnace process resulting in liquid pig iron, the main unit being the blast furnace, along with hot metal treatment units, blast furnace blowers, blast furnace hot stoves, compressed air production, steam injection in the blast furnace unit, steam generation plant, etc.;
- Emissions control – for treating releases to air, water or ground, including slag treatment, waste gas treatment, dedusting units, dust briquetting;
- Miscellaneous not covered above.
The diagram below shows the example of a large blast furnace within an integrated steel mill producing 5,000,000 tonnes of pig iron:
Please note that these values are purely for explanatory and illustrative purposes, as each factory will have its unique design
Inputs
CBAM Goods (Relevant Precursors):
- Internal Precursor:
- Sintered ore: 5,440,000 tonnes (1.088 tonnes/tonne of pig iron)
- Direct embedded emissions: 1,419,461.20 tCO2e
- Indirect embedded emissions: 268,924.86 tCO2e
- External Precursor:
- Pellets: 1,790,000 tonnes (0.358 tonnes/tonne of pig iron)
- Direct embedded emissions: 501,200 tCO2e
- Indirect embedded emissions: 89,500 tCO2e
Raw materials:
- Iron ore (Lump Ore): 900k tonnes (0.18 tonnes/tonne of pig iron)--> no GHG emissions
- Calcium carbonate (limestone): 128,500 tonnes (0.0257 tonnes/tonne of pig iron) --> 56,540 tCO2e
Fuels:
- Coking coal: 1,795,000 tonnes (0.359 tonnes/tonne of pig iron) --> 4,788,557.40 tCO2e
- Anthracite: 810,000 tonnes (0.162 tonnes/tonne of pig iron) --> 2,125,934.10 tCO2e
- Natural gas: 13,750,000 m3 --> 28,075.11 tCO2e
- Heavy fuel oil: 150,500 tonnes --> 470,607.48 tCO2e
- Coke oven gas: 12,222,222 m3 --> 8,793.18 tCO2e
Electricity:
In this example, the waste gas from the blast furnace (blast furnace gas) is used at a power plant located at the steel mill to produce part of the electricity. However, blast furnace gas, along with coke oven gas and basic oxygen furnace gas where applicable, is usually supplemented by purchased fuels such as oil and natural gas.
- Self-generated: 1M MWh
- Emission factor (calculated from the consumed natural gas and waste gas from the blast furnace): 0.8964 tCO2e/MWh
- Emissions: 268,920 tCO2e
- Grid: 100k MWh --> 22,830 tCO2e
Resulting in the following direct and indirect specific embedded emissions (SEE):
- Direct: 1.7230 tCO2e
- Indirect: 0.1987 tCO2e
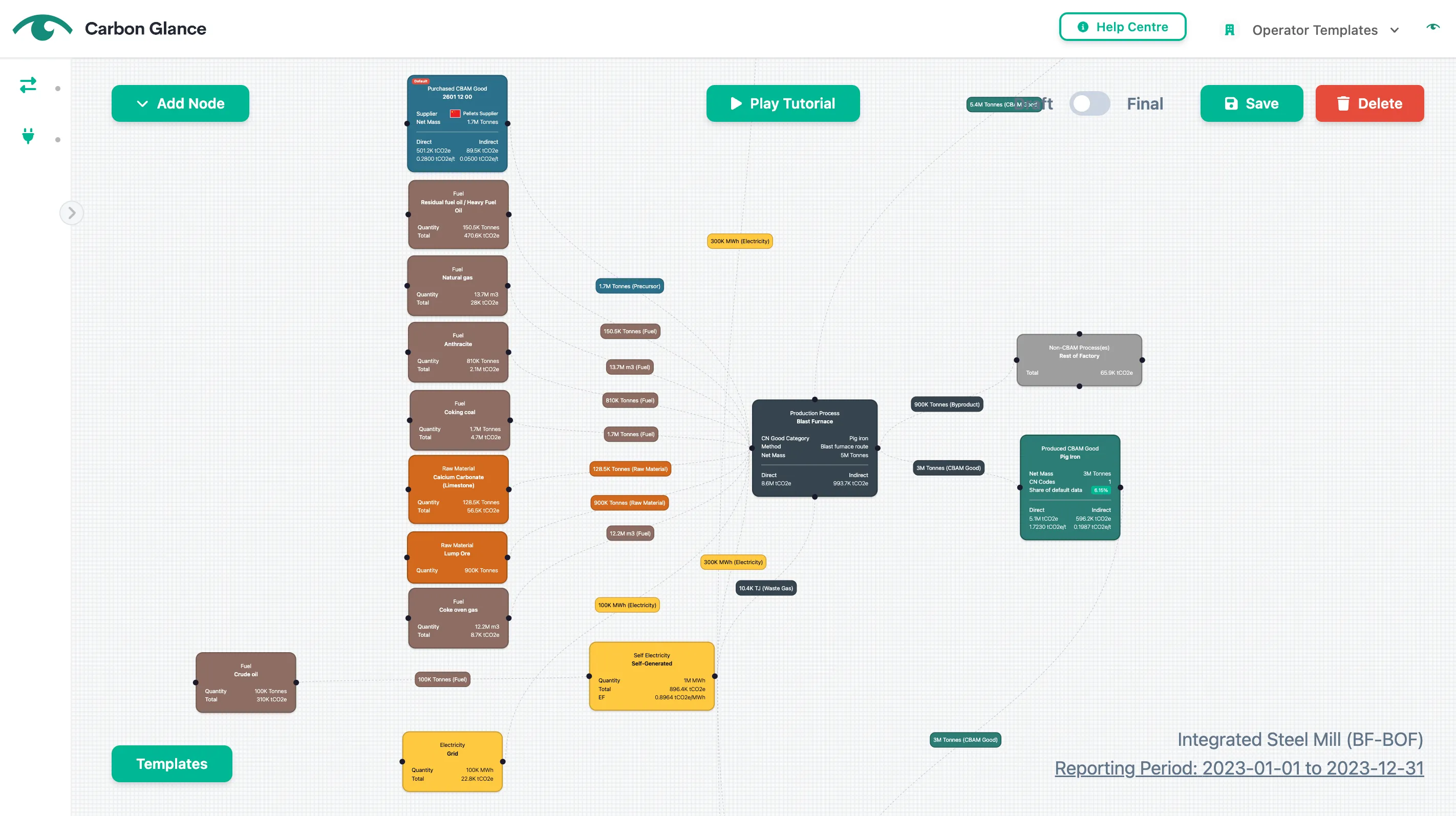
Smelting reduction processes, such as COREX and FINEX, offer a modern alternative to traditional blast furnace ironmaking. Both COREX and FINEX eliminate the need for coke ovens, significantly reducing the emissions associated with that process. Instead, non-coking coal can be used directly. Smelting-reduction technology enables a wide range of coals to be used.
FINEX, in particular, allows the use of fine iron ore without prior sintering. This streamlined process can result in lower emissions compared to COREX.
The smelting reduction process also generates molten pig iron and slag, and also waste gas. As with the Blast Furnace route, carbon remaining in the product, slag, or waste is taken into account using a mass balance method.
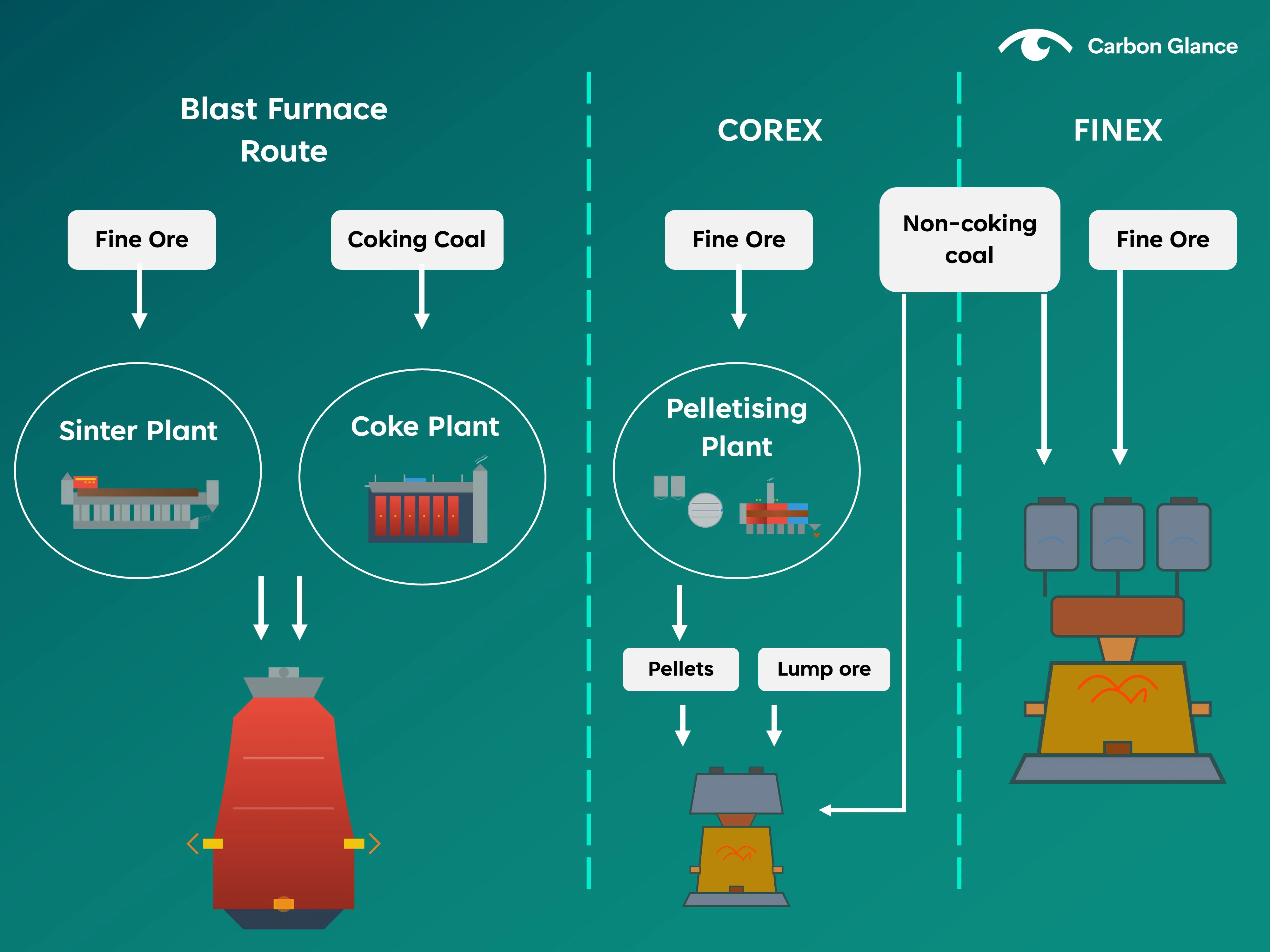
The EU CBAM covers the following production steps for the smelting reduction process:
- Raw material handling and pre-treatment
- Fuel storage and preparation
- Smelting reduction process - all steps for the smelting process, resulting in hot metal
- Casting plant
- Emissions control - in particular flue gas cleaning
Direct Reduced Iron (DRI) involves the reduction of iron ore to iron using a reducing gas (primarily carbon monoxide and hydrogen) at temperatures below the melting point of iron (less than 1,000°C). This occurs in a direct reduction reactor.
The main input materials used are:
- Iron ore (usually in the form of pellets or lumps due to its high iron content and shape)
- Reducing gas, which can be derived from natural gas, coal, and other fossil fuels (potentially hydrogen as well)
In natural gas-based DRI production, hydrogen does play a role in the reduction process, though this is in combination with carbon. Production routes using green or blue hydrogen may play a relevant role in decarbonising the steel industry in the future.
The main output material obtained is solid DRI, also known as sponge iron. The DRI is normally used as a replacement for scrap metal in the electric arc furnace steelmaking route. However, it may also be used as a feedstock for blast furnace iron making.
A drawback of DRI is that it can pose a fire hazard. Therefore, DRI can be melted into briquettes in a briquetting machine, as hot briquetted iron (HBI), when the product needs to be stored or transported over some distance.
DRI processes can be divided up by the type of reactor employed, namely:
- Shaft furnaces
- Rotary kilns
- Rotary hearth furnaces
- Fluidised bed reactor
Many of these solid-state processes use natural gas as the fuel and as the reducing agent (carbon monoxide and hydrogen).
The main benefit of a direct reduction unit compared to a blast furnace is that the direct reduction unit uses natural gas or coal as a fuel. Therefore, a coke oven plant is no longer needed, significantly reducing emissions.
An alternative to DRI is iron carbide. Iron carbide is also produced by means of a direct reduction and can be used in the same applications as DRI.
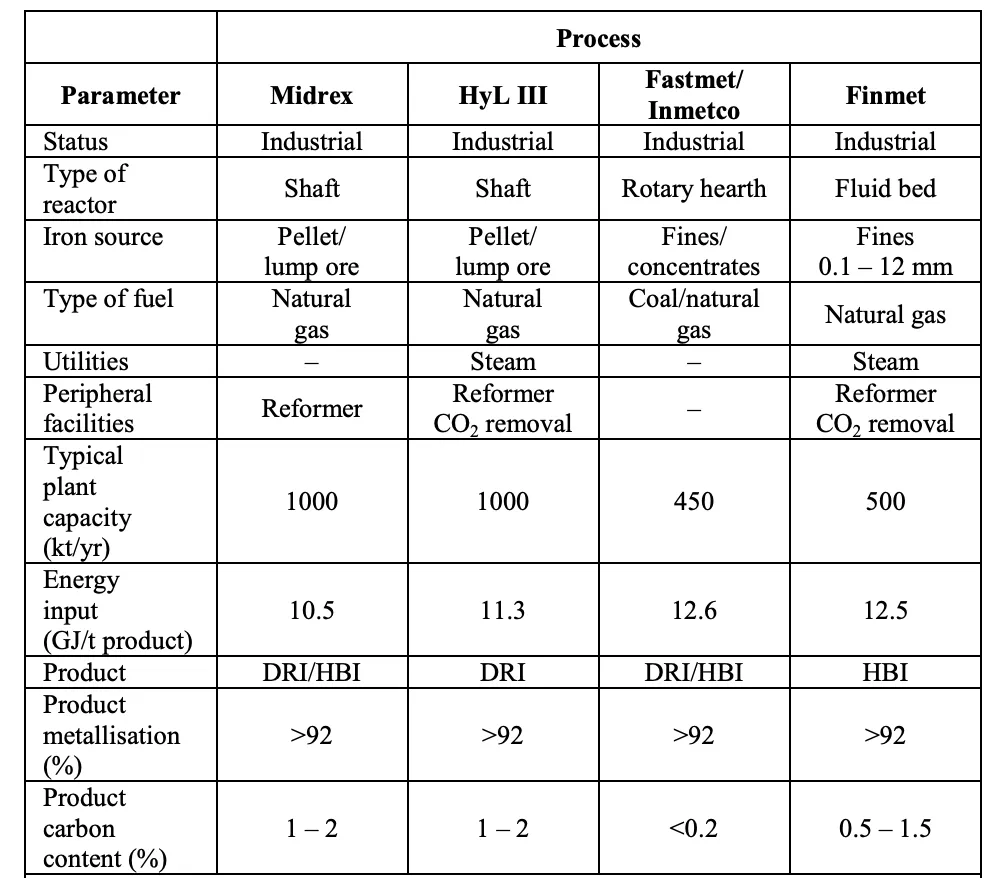
The EU CBAM covers the following production steps for the Direct Reduced Ironmaking (DRI):
- Raw material handling and pre-treatment
- Fuel storage and preparation - coal, natural gas or hydrogen
- Direct reduction process for iron production - all steps for the DRI process, forming into hot briquetted iron (HBI), if applicable
- Emissions control - in particular flue gas cleaning
The diagram below shows the example of an installation producing 1,000,000 tonnes of direct reduced iron (DRI):
Please note that these values are purely for explanatory and illustrative purposes, as each factory will have its unique design
Inputs
CBAM Goods (Relevant Precursors):
- External Precursor:
- Pellets: 1,050,000 tonnes
- Direct embedded emissions: 294,000 tCO2e
- Indirect embedded emissions: 52,500 tCO2e
Raw materials:
- Lump ore: 450,000 tonnes --> no GHG emissions
Fuels:
- Natural gas: 312,500,000 m3 --> 673,200 tCO2e
Intermediate goods:
- Recycled Pelletized Dust: 25k tonnes --> no GHG emissions
Electricity:
- Grid: 110,000 MWh → 64,196 tCO2e (indirect emissions)
Resulting in the following direct and indirect specific embedded emissions (SEE):
- Direct: 0.9672 tCO2e/tonne
- Indirect: 0.1167 tCO2e/tonne
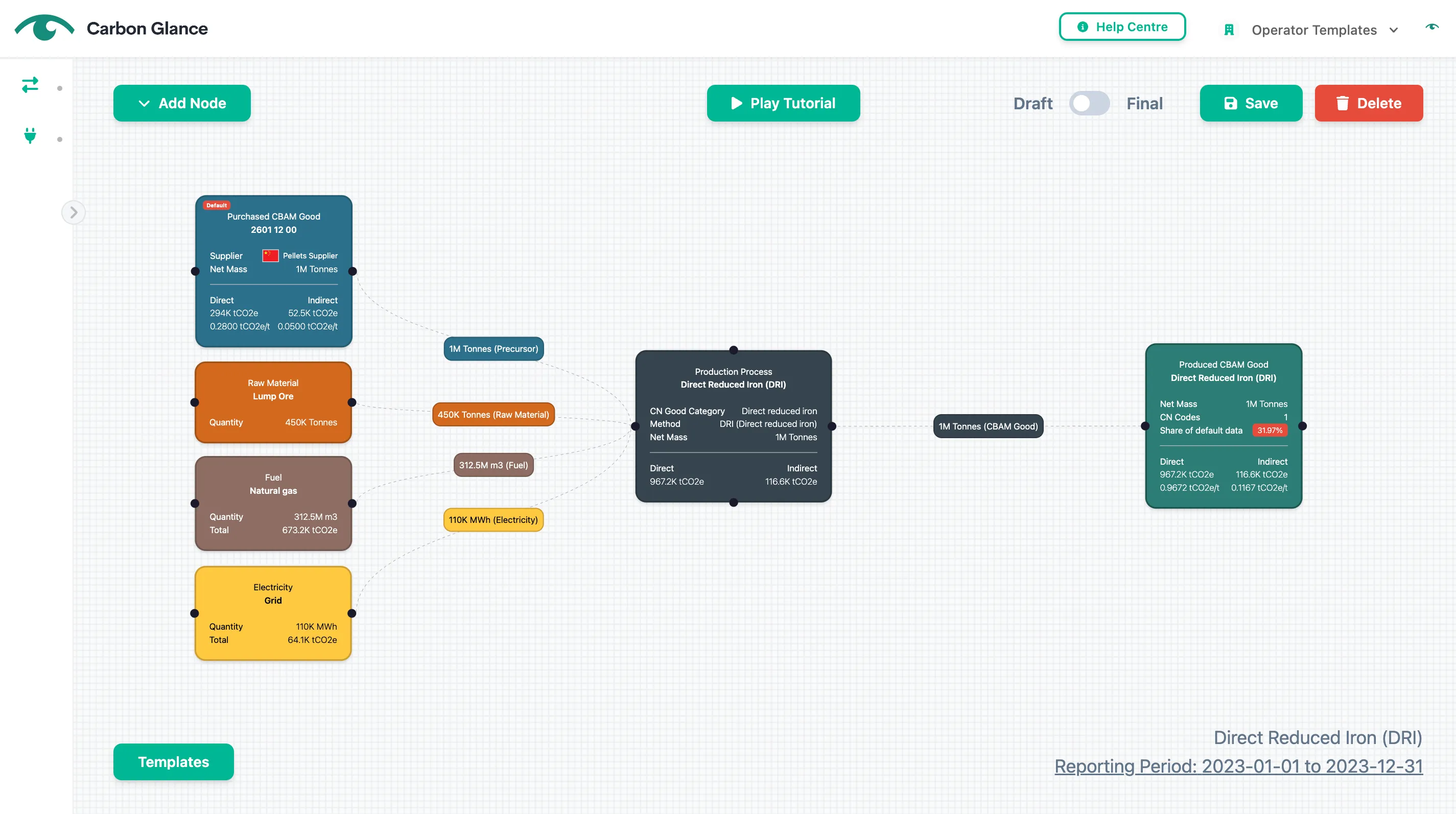
1️⃣ CBAM Reminder: Only primary hot-rolling and rough shaping by forging to obtain the semi-finished products under CN codes 7207, 7218, and 7224 are included in this aggregated goods category (Crude Steel).
All other rolling and forging processes are included in the aggregated goods category "iron or steel products".
2️⃣ CBAM Reminder: Carbon entering the process in scrap, alloys, and graphite, and carbon remaining in the product or in slags or wastes is taken into account by using the mass balance method.
If the basic oxygen steelmaking production route starts with hot metal (liquid pig iron), the hot metal is directly converted to crude steel by the basic oxygen converter or furnace (BOF) as part of a continuous process.
Following the BOF, a steel decarburisation process by argon oxygen decarburisation (AOD) or vacuum oxygen decarburisation (VOD) may be performed if further refinement of the steel's carbon content is needed. This usually happens when producing certain grades of steel that require low carbon content for specific applications, such as high-strength steels, stainless steels, or other specialty alloys. These steels demand a precise control of carbon to achieve the desired mechanical properties or corrosion resistance.
AOD: In this process, oxygen is blown into the steel along with argon gas. The argon helps to control the flow of gases, ensuring that the oxygen is used more effectively. This process is typically used for producing stainless steels and other special alloys.
VOD: In this process, the decarburization happens in a vacuum. A vacuum is applied to the steel, which reduces the pressure in the vessel. This method is typically used for making high-quality steels that require a very low carbon content, such as those used in aerospace or medical applications.
These processes are followed by various secondary metallurgical processes such as vacuum degassing to remove dissolved gases like hydrogen and nitrogen. The crude steel is then cast into its primary forms by continuous casting or ingot casting, which may be followed by hot-rolling or forging to obtain the semi-finished crude steel products.
The gas generated during the BOF process consists mainly of carbon monoxide. The gas is cleaned or scrubbed in the cleaning plant. After BOX gas is mixed with BF gas, it if burnt in the rolling mill or combined heat and power (CHP) plants.
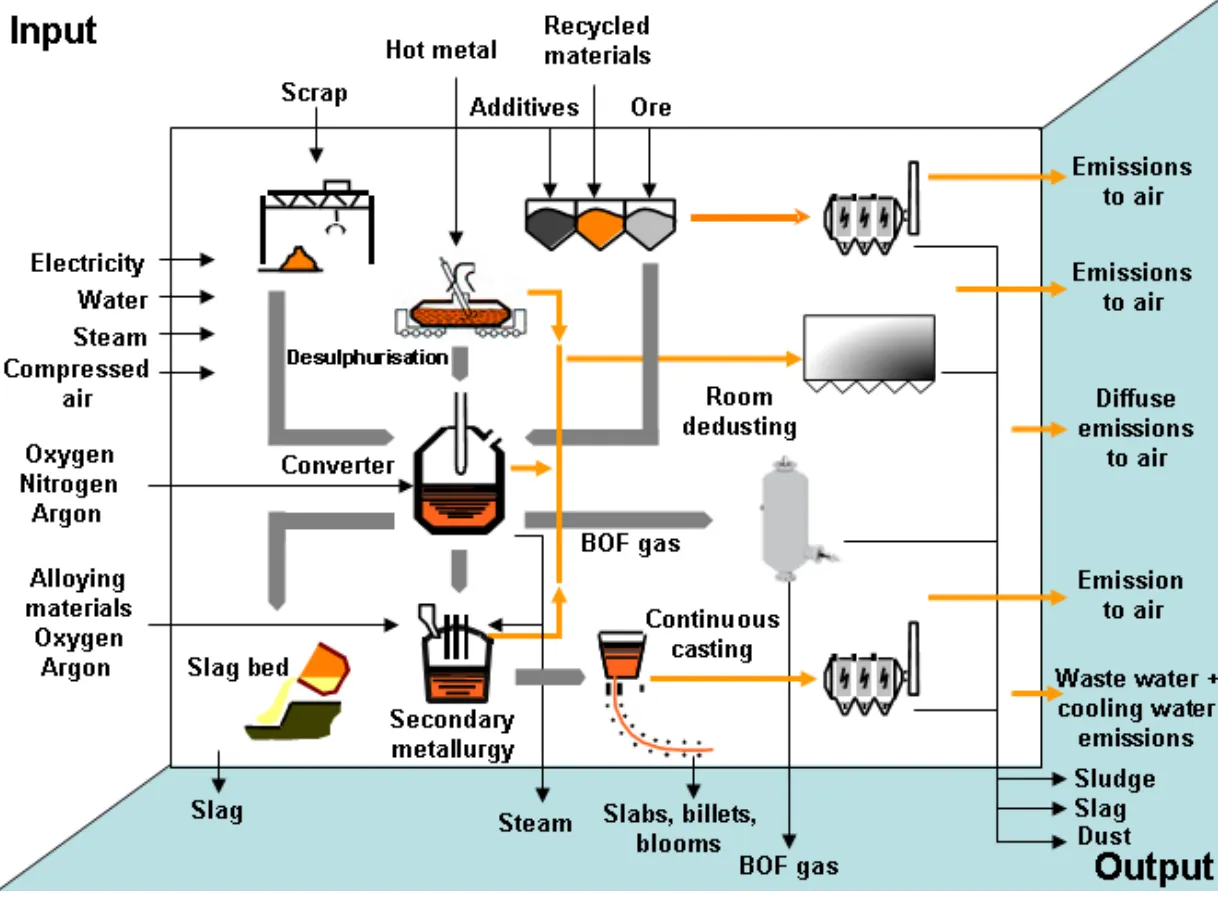
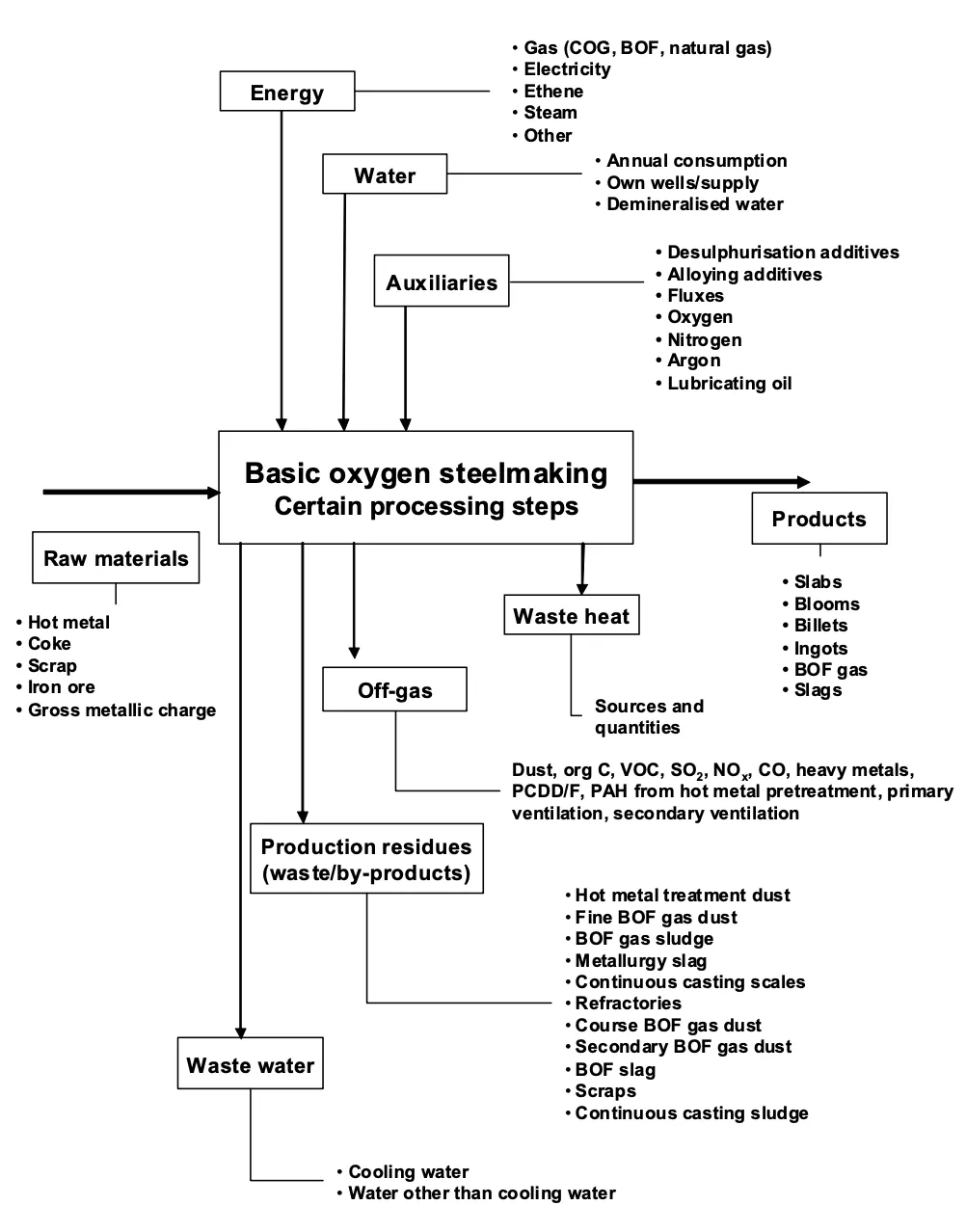
The EU CBAM covers the following production steps for crude steel:
- Basic oxygen converter or furnace (BOF)
- Decarburisation - AOD or VOD processes, where relevant
- Secondary metallurgy and vacuum degassing
- Casting plant - continuous casting or ingot casting, preheating equipment
- Hot rolling or forging - where relevant, only primary hot-rolling and rough shaping by forging to obtain the semi-finished products
- All necessary auxiliary activities - such as transfers, re-heating
- Emissions control - in particular flue gas cleaning, dedusting units, slag handling
The direct smelting of materials which contain iron is usually performed in an electric arc furnace (EAF). Feedstocks for EAF routes are metallic iron, in particular ferrous scrap and/or direct reduced iron (DRI). The ferrous scrap may be comprised of scrap from inside the steelworks, cut-offs from steel product manufacturers (e.g. vehicle builders) and capital or post-consumer scrap.
The feedstock is melted using electrical energy imparted to the charge through carbon electrodes and then refined and alloyed to produce the desired grade of steel. Following EAF smelting, a steel decarburisation process by argon oxygen decarburisation (AOD) or vacuum oxygen decarburisation (VOD) may be performed, followed by various secondary metallurgical processes such as desulphurisation and vacuum degassing to remove dissolved gases.
As in the BOF, a slag is formed from lime to collect undesirable components in the steel.
EAFs are typically stand-alone operations because of their fundamental reliance on scrap and not iron as a raw material. However, they can also be located in integrated plants.
CBAM Reminder: Only primary hot-rolling and rough shaping by forging to obtain the semi-finished products under CN codes 7207, 7218, and 7224 are included in this aggregated goods category (Crude Steel).
All other rolling and forging processes are included in the aggregated goods category "iron or steel products".
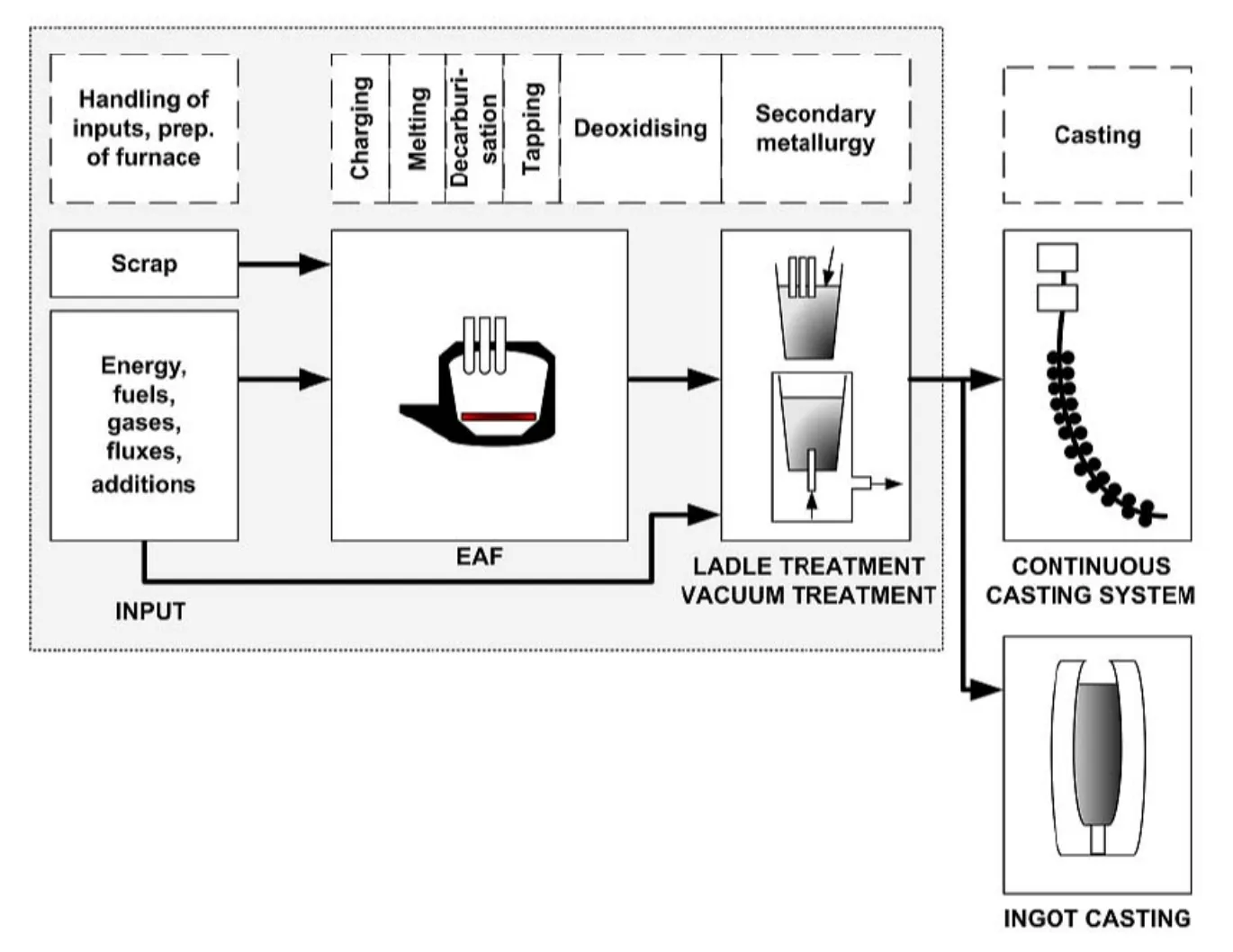
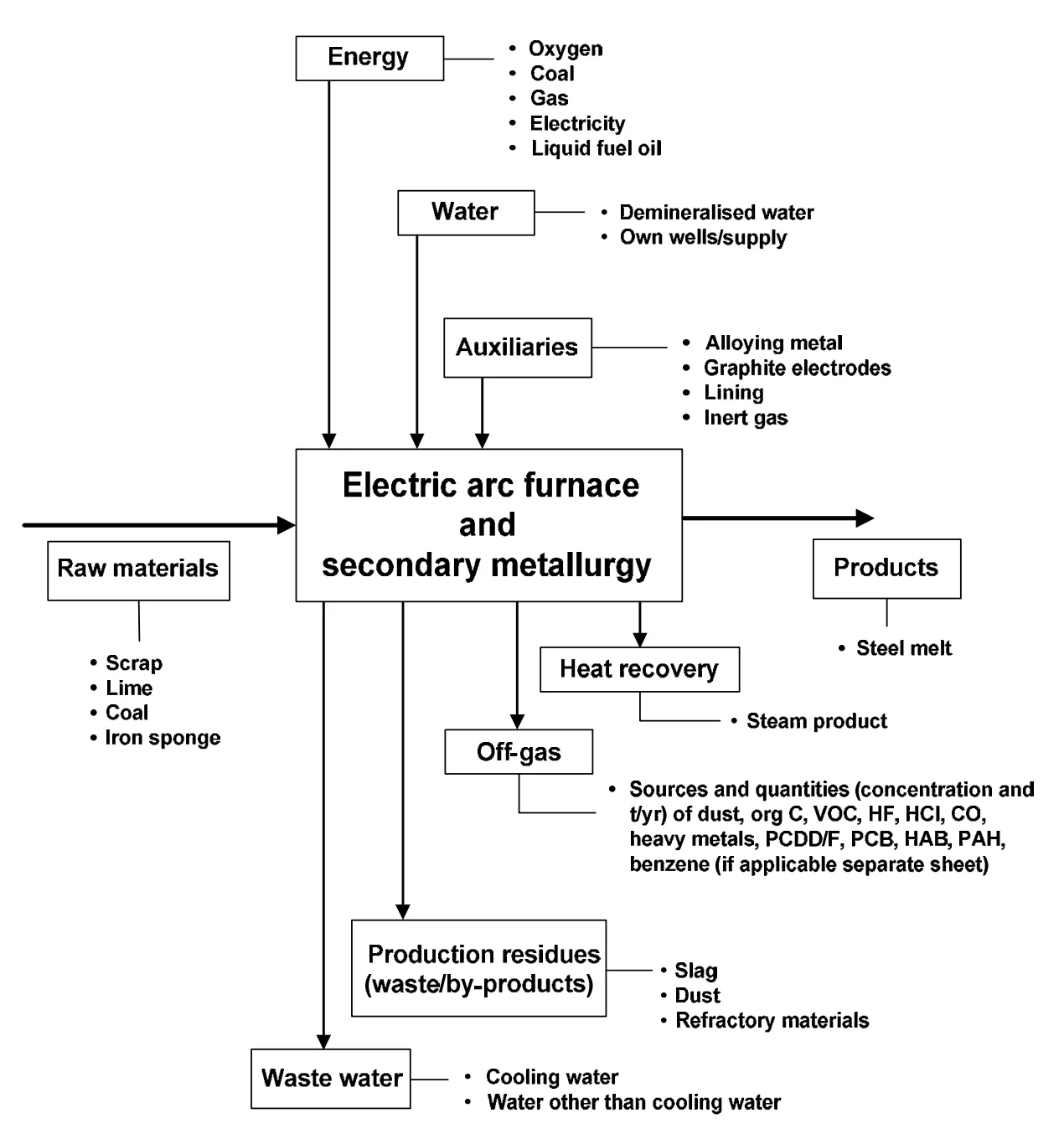
The EU CBAM covers the following production steps for the EAF route:
- Raw material handling and pre-treatment - scrap drying and pre-heating of raw materials
- EAF process - all steps for the EAF process, including charging, melting, primary refining and steel and slag tapping of the primary furnace
- Decarburisation - AOD or VOD processes, where relevant
- Secondary metallurgy and vacuum degassing
- Casting plant - continuous casting or ingot casting, pre-heating equipment
- Hot rolling or forging - where relevant, only primary hot-rolling and rough shaping by forging to obtain the semi-finished products
- All necessary auxiliary activities, such as transfers, heating of equipment, re-heating
- Emissions control - in particular flue gas cleaning, dedusting units, slag handling
Once the final steel quality has been achieved, the steel is conveyed in a casting ladle to the casting machines for casting. Casting products are subsequently processed in rolling mills and product finishing lines in order to prepare them for the market.
CBAM Reminder: Only primary hot-rolling and rough shaping by forging to obtain the semi-finished products under CN codes 7207, 7218, and 7224 are included in this aggregated goods category (Crude Steel).
All other rolling and forging processes are included in the aggregated goods category "iron or steel products".
Continuous Casting: Continuous casting is a process which enables the casting of one or a sequence of ladles of liquid steel into a continuous strand of billet, bloom, slab, beam blank or strip.
- Slabs: Slabs are semi-finished, continuous cast products for the rolling of flat products. These include thin slabs.
- Billets and Blooms: These are semi-finished continuous cast products used for the rolling of long products.
- Beam blanks: Beam blanks are continuously cast to near-net-shape. They are used for rolling of long products.
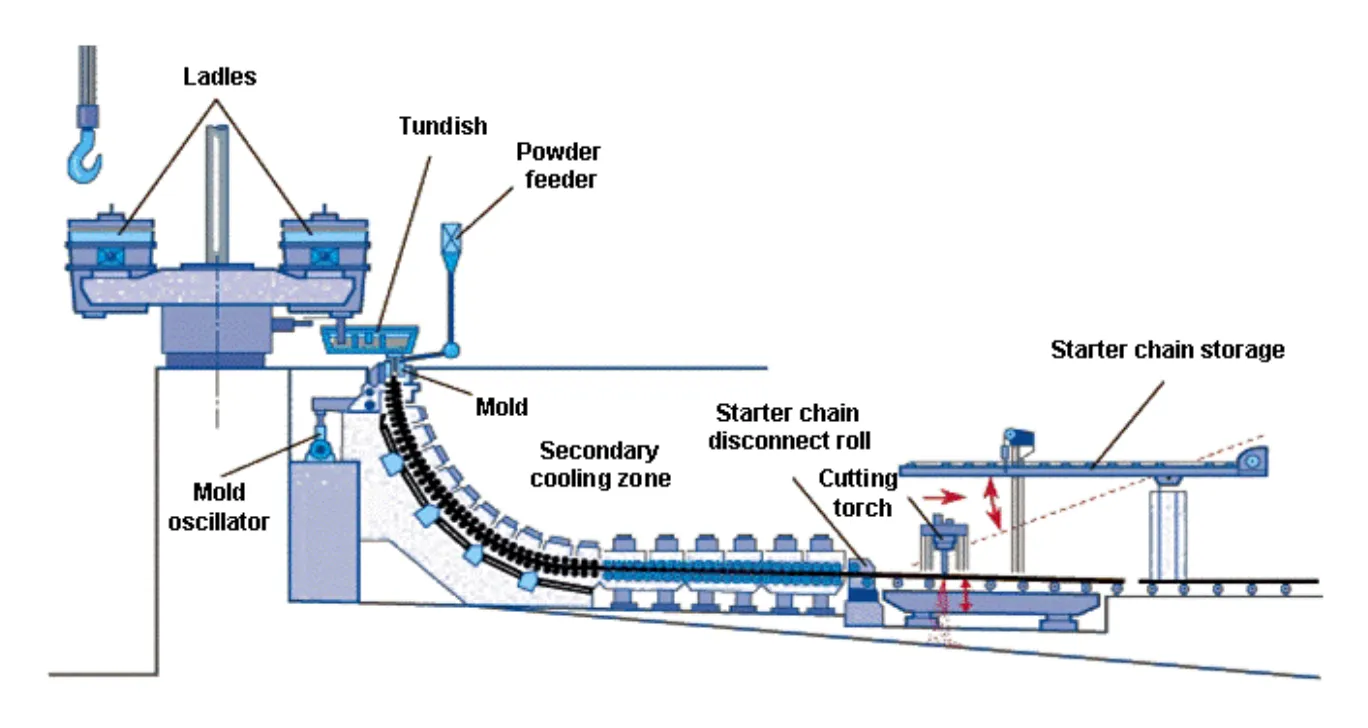
Ingot Casting: Ingot casting is a traditional, batch-based process where molten steel is poured into stationary molds (ingot molds) and allowed to solidify into large blocks called ingots.
After cooling, the ingots are taken out of the casting mould and transported to the rolling mills (blooming/slabbing mills). Subsequently, after preheating, the ingots are rolled into slabs, blooms or billets. In many places, ingot casting has been replaced by continuous casting. At least in the EU, only a minor part is cast into discrete moulds to produce ingots for some special products (e.g., heavy plates).
1️⃣ CBAM Reminder: If you produce final iron or steel products that contain more than 5% by mass of other materials (e.g., insulation materials in CN code 7309 00 30), only the mass of iron or steel shall be reported as the mass of the goods produced.
2️⃣ CBAM Reminder: The quantity of scrap generated during the production process must be excluded from the quantity produced.
The EU CBAM covers the following production steps for iron or steel products:
- Raw material preparation - including pre-heating and alloying
- Forming processes for basic steel products - all forming process steps, including casting, hot and cold rolling, shaping by forging, wire drawing
- Finishing activities - all finishing steps including surface treatment (such as pickling, annealing, plating, coating, galvanizing) and further fabrication (cutting, welding, finishing)
- Emissions control - for treating releases to air, water or ground
In hot rolling, the size, shape, and metallurgical properties of steel slabs, blooms, billets, or ingots are altered by repeatedly compressing the heated metal between electrically powered rollers. The steel input varies in form and shape, depending on the process route and the desired product. For hot rolling, the steel must be heated to temperatures between 1,050°C and 1,300°C. Depending on the charge material and the hot rolling process, reheating is done in different types of furnaces, which are usually fired by oil, natural gas or iron and steel process gases such as coke oven gas (COG) and blast furnace gas (BFG).
Slabs, blooms, and billets can exhibit surface defects such as cracks, folds, or seams. Therefore, before further processing, it might be necessary to ensure the rolled product has flawless surfaces through methods like scarfing, grinding, or cutting.
Hot rolling mills are classified by the type of product that they produce and by their design and features. These include:
- Blooming/Slabbing mills: Blooming and slabbing mills are utilized to roll cast ingots into semi-finished products, such as blooms and slabs, which are then further processed in other mills. With the advancement and widespread adoption of continuous casting technology, the prevalence of these mills has significantly decreased.
- Hot strip mills: Hot strip mills transform heated slabs into steel strips through a series of rolling processes. Hot-rolled strip not intended for further processing in cold mills is sometimes sold with rolled edges. However, depending on the intended application, the strip may require additional conditioning.
- Plate mills: Plate mills are dedicated to producing steel plates of varying thicknesses and dimensions. Plate mills typically begin with reheating thick steel slabs to a malleable temperature. These slabs are then passed through a series of rolling stands to achieve the desired thickness and width.
- Bar and rod mills: Bar and rod mills specialise in the production of metal bars and rods (wire rods) through rolling processes. These mills transform metal billets or ingots into various profiles and sizes. Hot rolled wire rod is mainly used for further processing in cold drawing or cold rolling.
- Structural/Section mills: Structural/Section mills are dedicated to producing long steel products with specific cross-sectional profiles, such as beams, channels, angles, and other structural shapes. Like most other types of mills, section rolling mills are divided into roughing, intermediate and finishing stands, preceded by a descaling machine installed between the reheating furnace and the roughing mill.
- Tube mills: Tube mills are designed for the production of metal tubes and pipes through various forming and welding processes
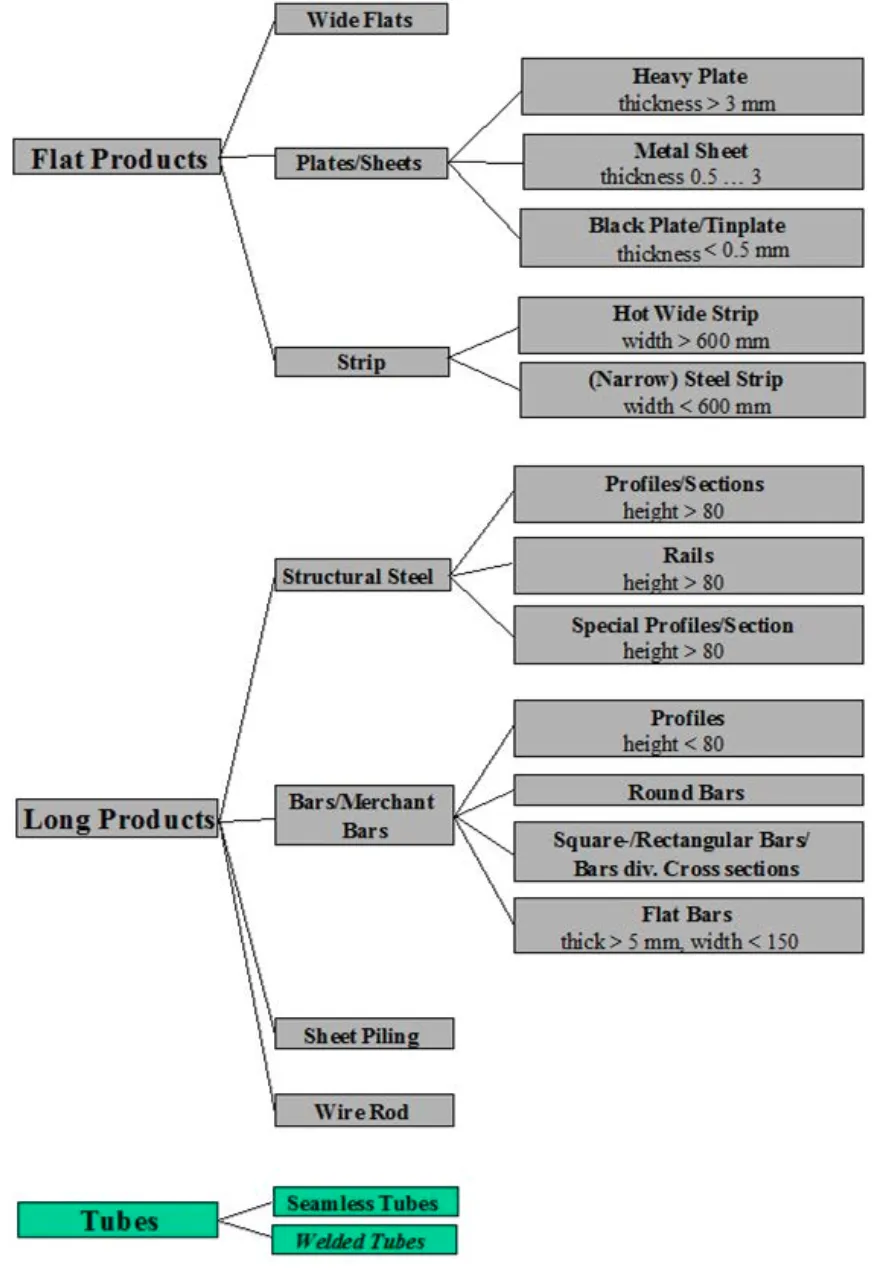
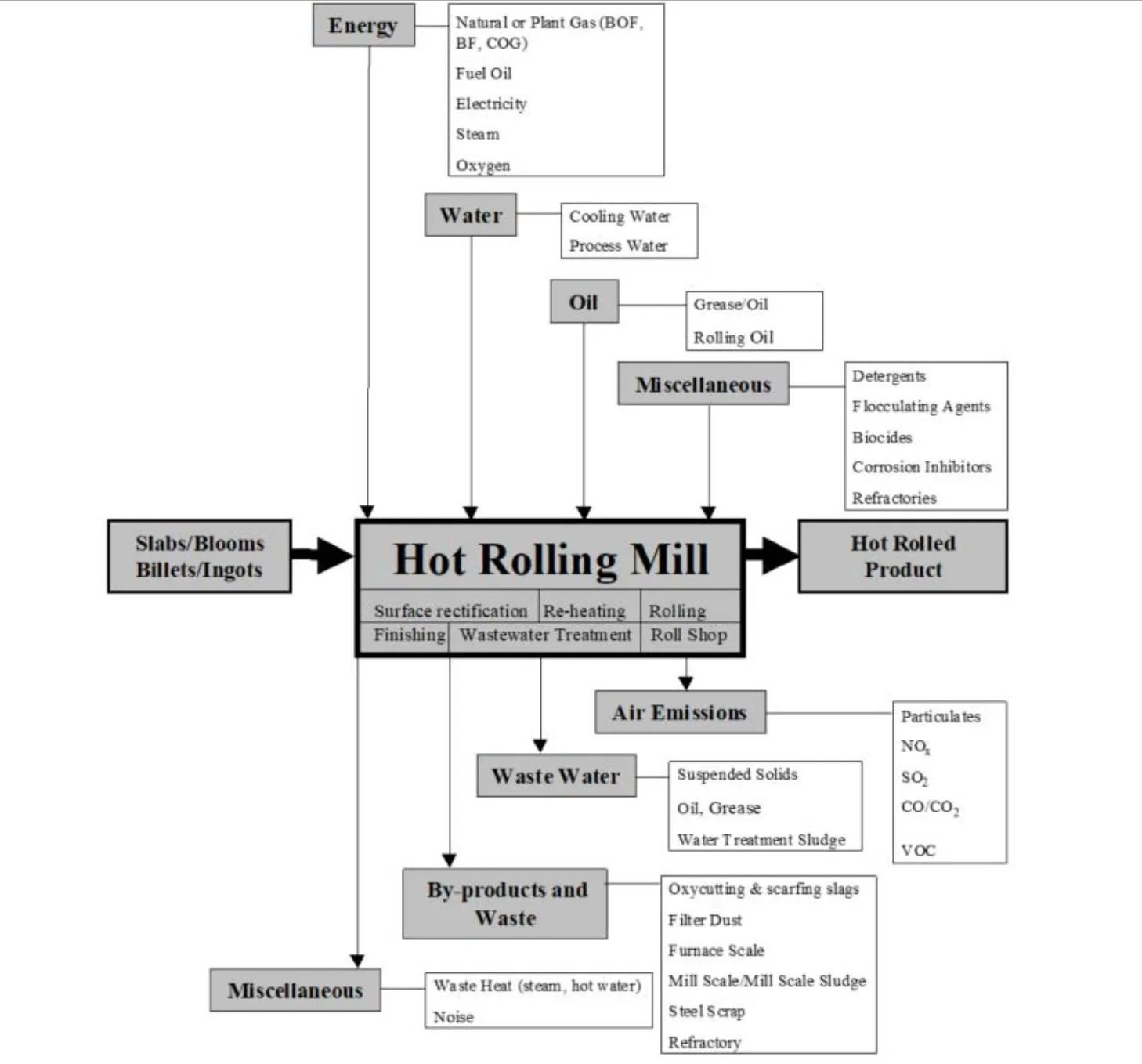
The diagram below shows the example of hot rolling mill producing 50,000 tonnes of finished steel products:
Please note that these values are purely for explanatory and illustrative purposes, as each factory will have its unique design
Inputs
CBAM Goods (Relevant Precursors):
- External Precursor: Semi-finished products of non-alloy free-cutting steel
- Flat-rolled products (hot-rolled): 55,000 tonnes
- Direct embedded emissions: 108,900 tCO2e
- Indirect embedded emissions: 3,850 tCO2e
Fuels:
- Natural gas: 2,718,246 m3 --> 5,550.19 tCO2e
Electricity:
- Grid: 10,000 MWh --> 2,283 tCO2e
Resulting in the following direct and indirect specific embedded emissions (SEE):
- Direct: 2.2890 tCO2e/tonne
- Indirect: 0.1227 tCO2e/tonne
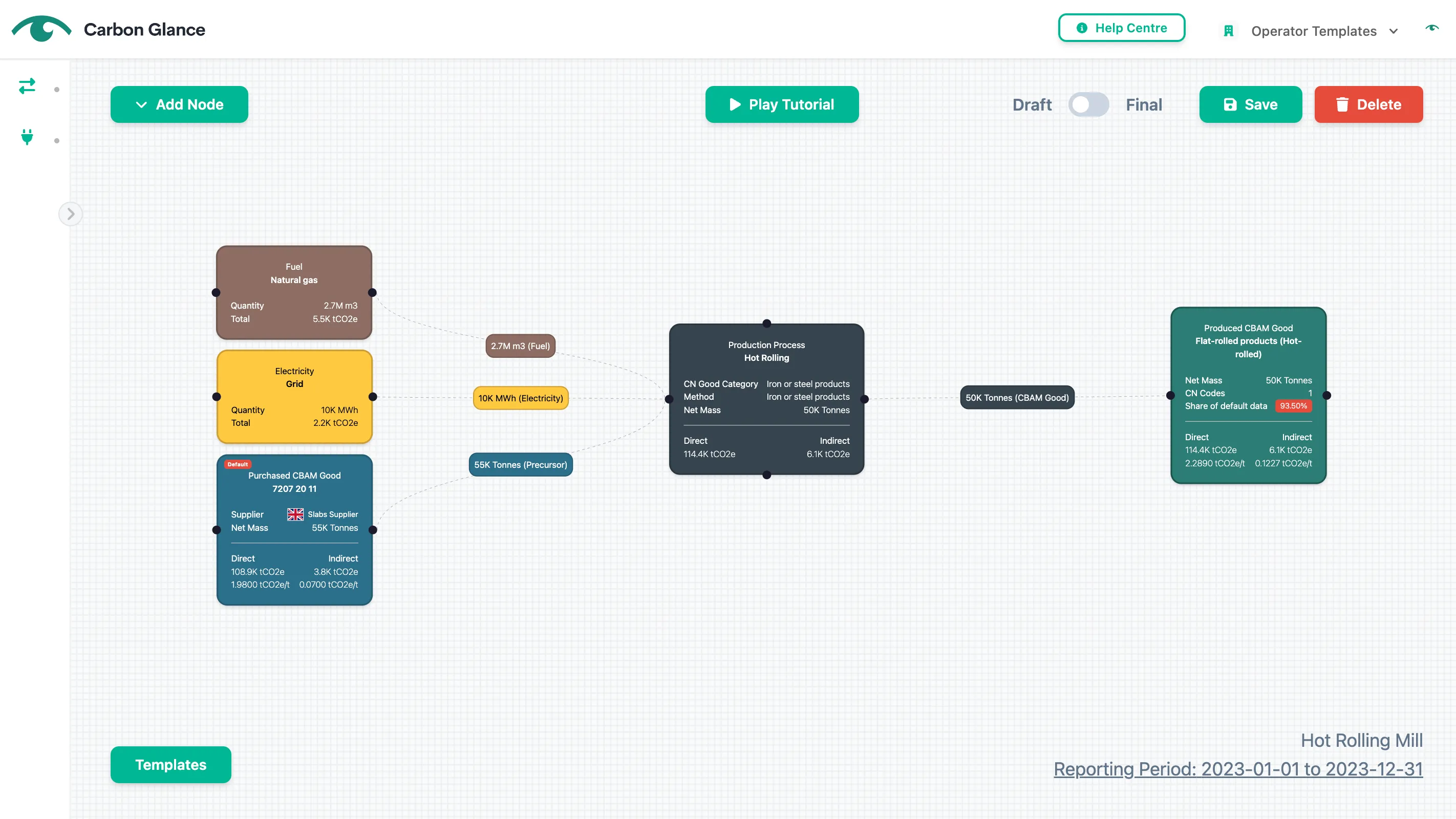
The primary purpose of cold rolling steel is to reduce the thickness of hot-rolled steel strips into thinner gauges. Beyond thickness reduction, cold rolling enhances surface finish, improves thickness tolerances, offers a range of tempers, enhances physical characteristics, and prepares the strip for surface coating.
In cold rolling, the properties of hot-rolled strip products are modified by compressing the material between rollers, typically without prior heating. In some cases, prior annealing of the hot strip may be performed. The input material is supplied in coil form from hot rolling mills.
The processing steps and their sequence in a cold rolling mill depend on the quality of the steel being processed. For low-alloy and alloy steels (carbon steels), the typical sequence is: pickling, rolling, annealing, temper rolling/skin pass rolling, and finishing.
In contrast, processing high-alloy steels (stainless steels) requires an initial annealing step before pickling due to the material's hardness. Additionally, multiple annealing and/or pickling steps may be necessary during rolling.
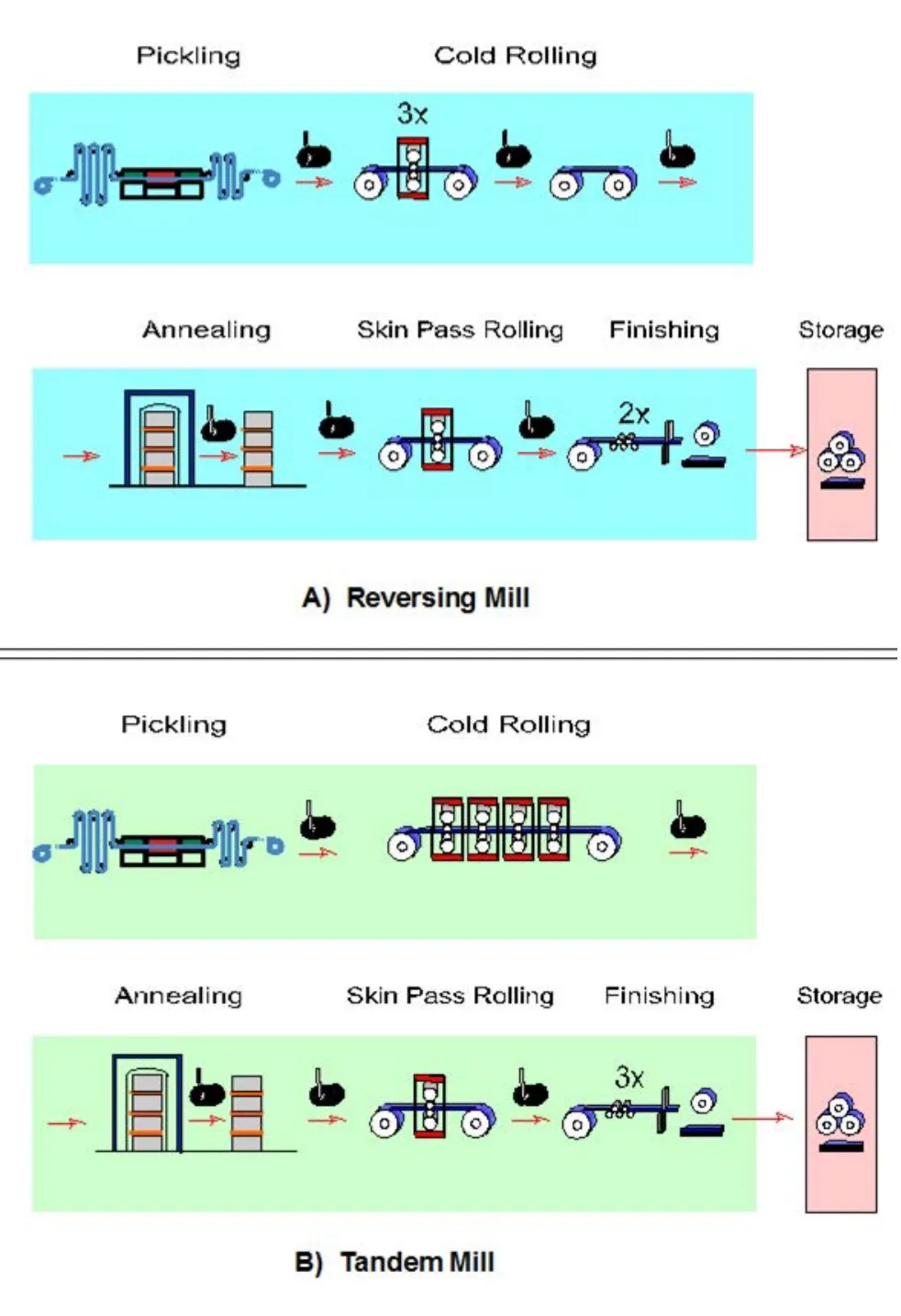
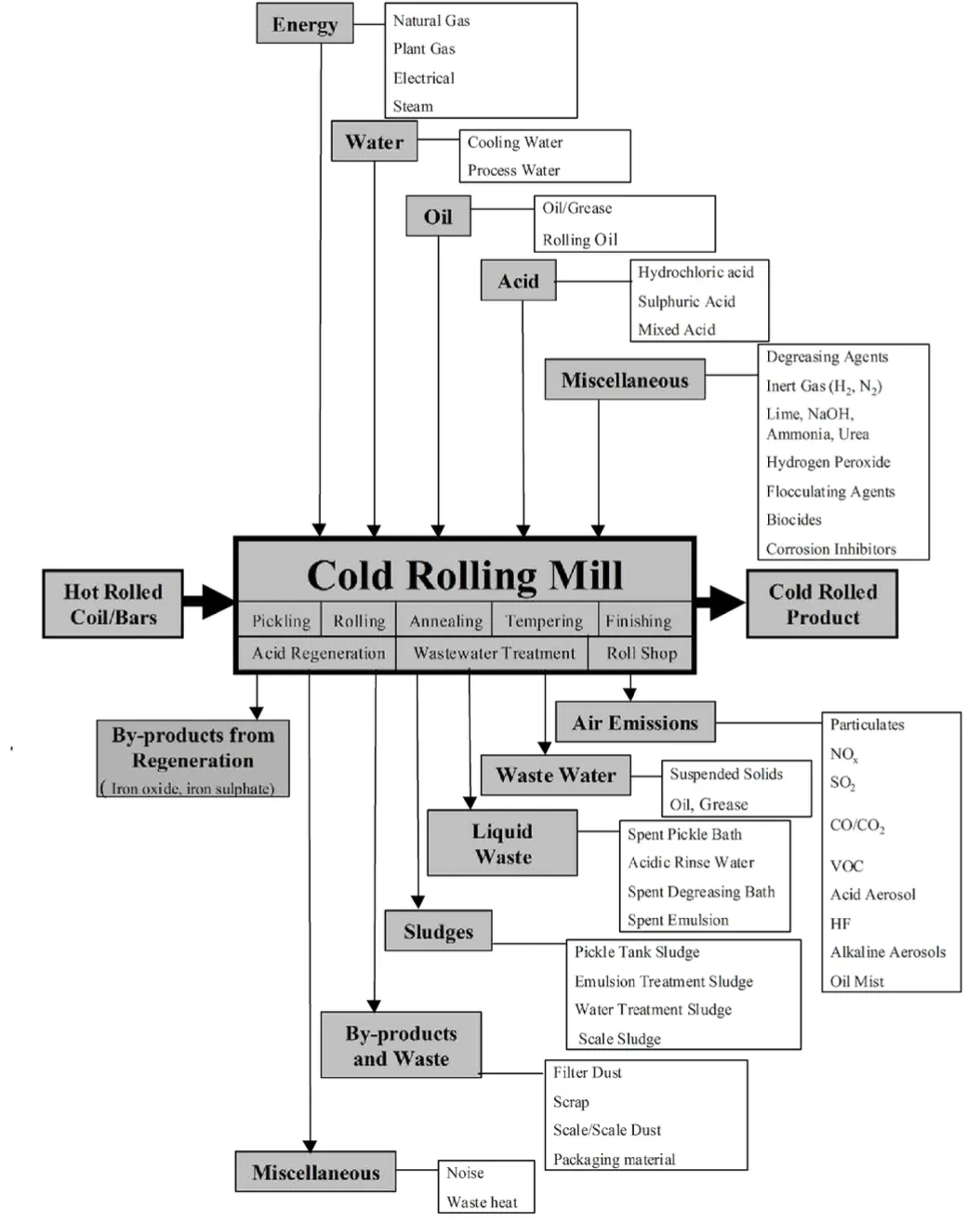
The diagram below shows the example of cold rolling mill producing 50,000 tonnes of finished steel products:
Please note that these values are purely for explanatory and illustrative purposes, as each factory will have its unique design
Inputs
CBAM Goods (Relevant Precursors):
- External Precursor:
- Flat-rolled products (hot-rolled): 50,000 tonnes
- Direct embedded emissions: 101,500 tCO2e
- Indirect embedded emissions: 4,000 tCO2e
Electricity (Note that in this example, some of the total electricity consumed by the factory is used for non-CBAM processes and, hence, is not included in the CBAM emissions calculations):
- Grid: 19,500 MWh:
- CBAM Emissions: 4,109.4 tCO2e (indirect emissions)
- Non-CBAM emissions: 342.45 tCO2e
Adding the non-CBAM emissions helps corroborate the emissions associated with the total electricity consumption of the factory and ensures that all electricity emissions have been fully and correctly attributed.
Resulting in the following direct and indirect specific embedded emissions (SEE):
- Direct: 2.0300 tCO2e/tonne
- Indirect: 0.1622 tCO2e/tonne
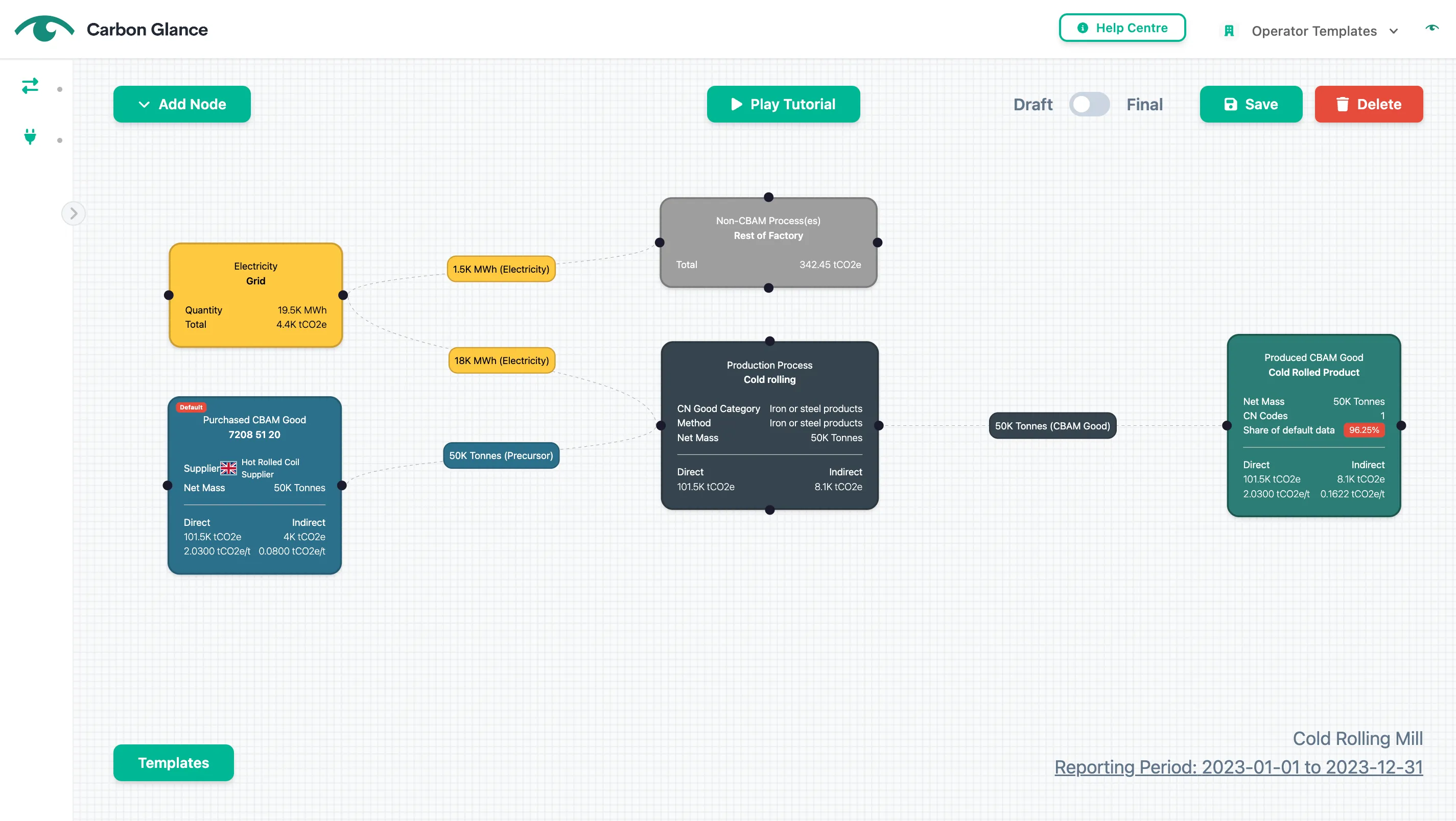
The diagram below illustrates the same example as above; however, this time, 25k tonnes of the cold-rolled products are sent to a separate plant for galvanizing and then returned:
According to the EU CBAM, if some processes occur in secondary installations or are outsourced to other companies and the goods are subsequently returned to the primary installation, they may be treated as if they happen in the primary installation.
Please note that these values are purely for explanatory and illustrative purposes, as each factory will have its unique design
Inputs
CBAM Goods (Relevant Precursors):
- External Precursor:
- Flat-rolled products (hot-rolled): 50,000 tonnes
- Direct embedded emissions: 101,500 tCO2e
- Indirect embedded emissions: 4,000 tCO2e
Fuels (the quantity of fuel used should be provided by the operator of the installation where galvanasing took place):
- Natural gas: 525,000 m3 --> 1,071.96 tCO2e
Electricity (Note that in this example, some of the total electricity consumed by the factory is used for non-CBAM processes and, hence, is not included in the CBAM emissions calculations):
- Internal:
- Grid: 19,500 MWh:
- CBAM Emissions: 4,109.4 tCO2e (indirect emissions)
- Non-CBAM emissions: 342.45 tCO2e
- External (the electricity consumed by the factory where galvanising took place, which needs to be collected from that operator's plant): 13,800 MWh --> 3,150.54 tCO2e (indirect emissions)
Resulting in the following direct and indirect specific embedded emissions (SEE):
- Cold Rolled Products:
- Direct: 2.0300 tCO2e/tonne
- Indirect: 0.1622 tCO2e/tonne
- Galvanized Cold rolled Products:
- Direct: 2.0729 tCO2e/tonne
- Indirect: 0.2882 tCO2e/tonne
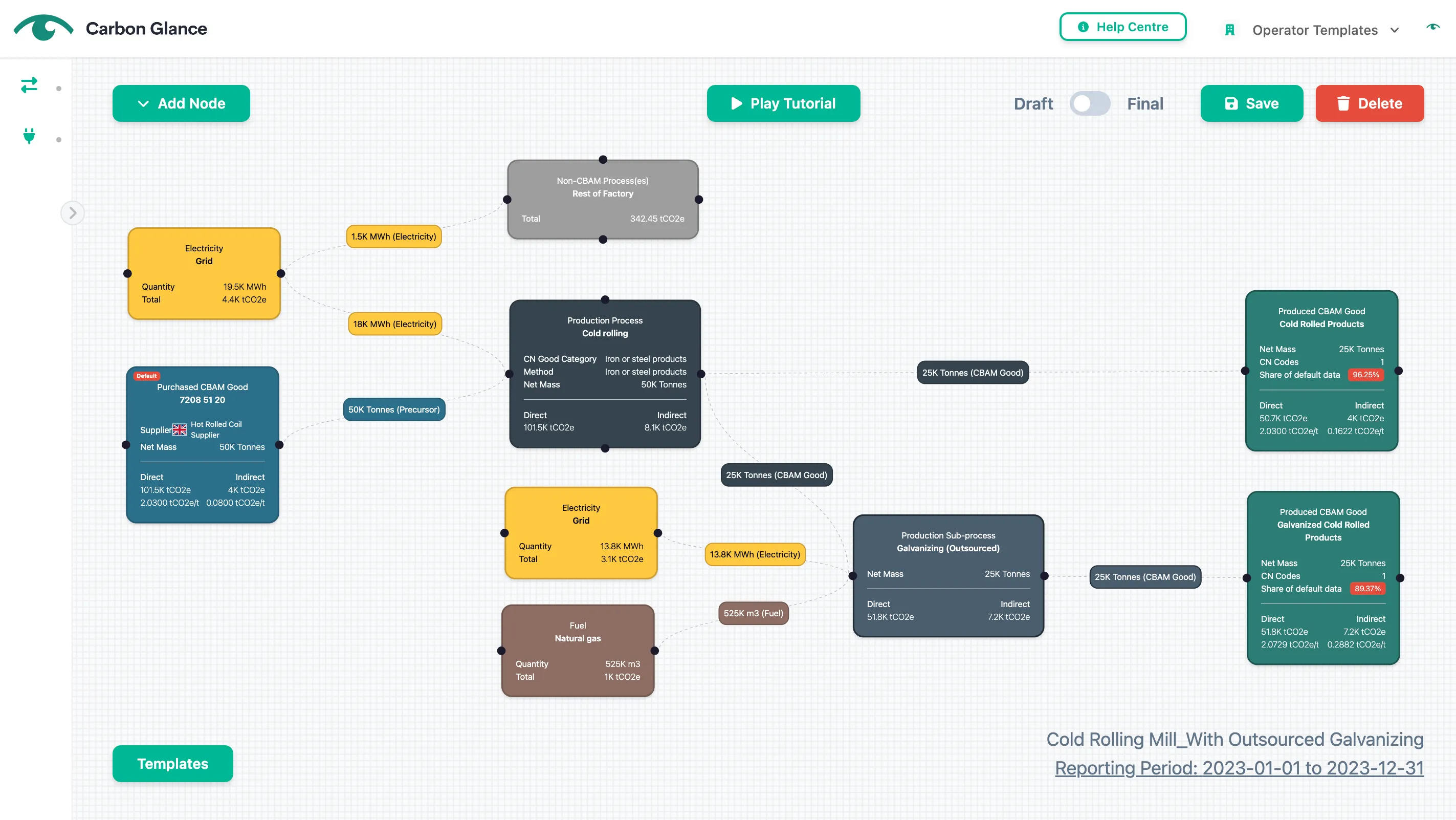
Wire drawing is a process in which wire rods/wires are reduced in size by drawing them through cone-shaped openings of a smaller cross section, so-called dies.
The input is usually wire rod from hot rolling mills in the form of coils. A typical wire drawing process line comprises the following steps:
- Pre-treatment of the wire rod (mechanical descaling, pickling);
- Dry or wet drawing (usually several drafts with decreasing die sizes);
- Heat treatment (continuous/discontinuous annealing, patenting, oil hardening)
- Finishing.
Wire is manufactured in different grades of steel (low-carbon steel, high-carbon steel, stainless steel and other alloy steel). Wire is sent in coil form to further processing, like coating and manufacturing of other finished products.
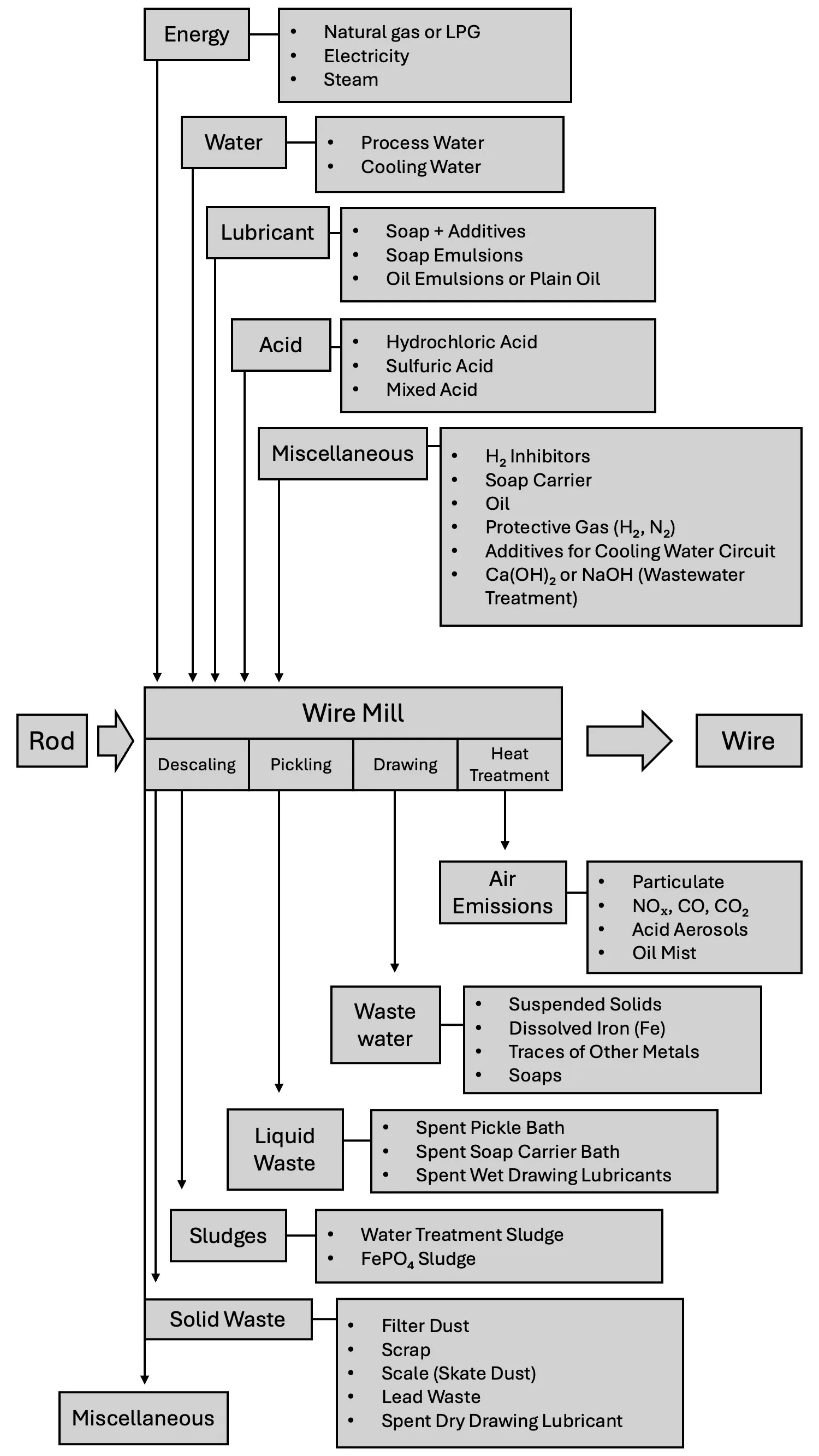
In the hot dip coating process, the steel is continuously passed through molten metal. An alloying reaction between the two metals takes place, leading to a good bond between the coating and the substrate.
Metals suitable for use in hot dip coating are those which have a melting point low enough to avoid any thermal changes in the steel product, for example aluminium, lead and zinc. Continuous hot dip coating is commonly used for steel sheets and strips that are later fabricated into products like automotive body panels, appliances, and HVAC ducting.
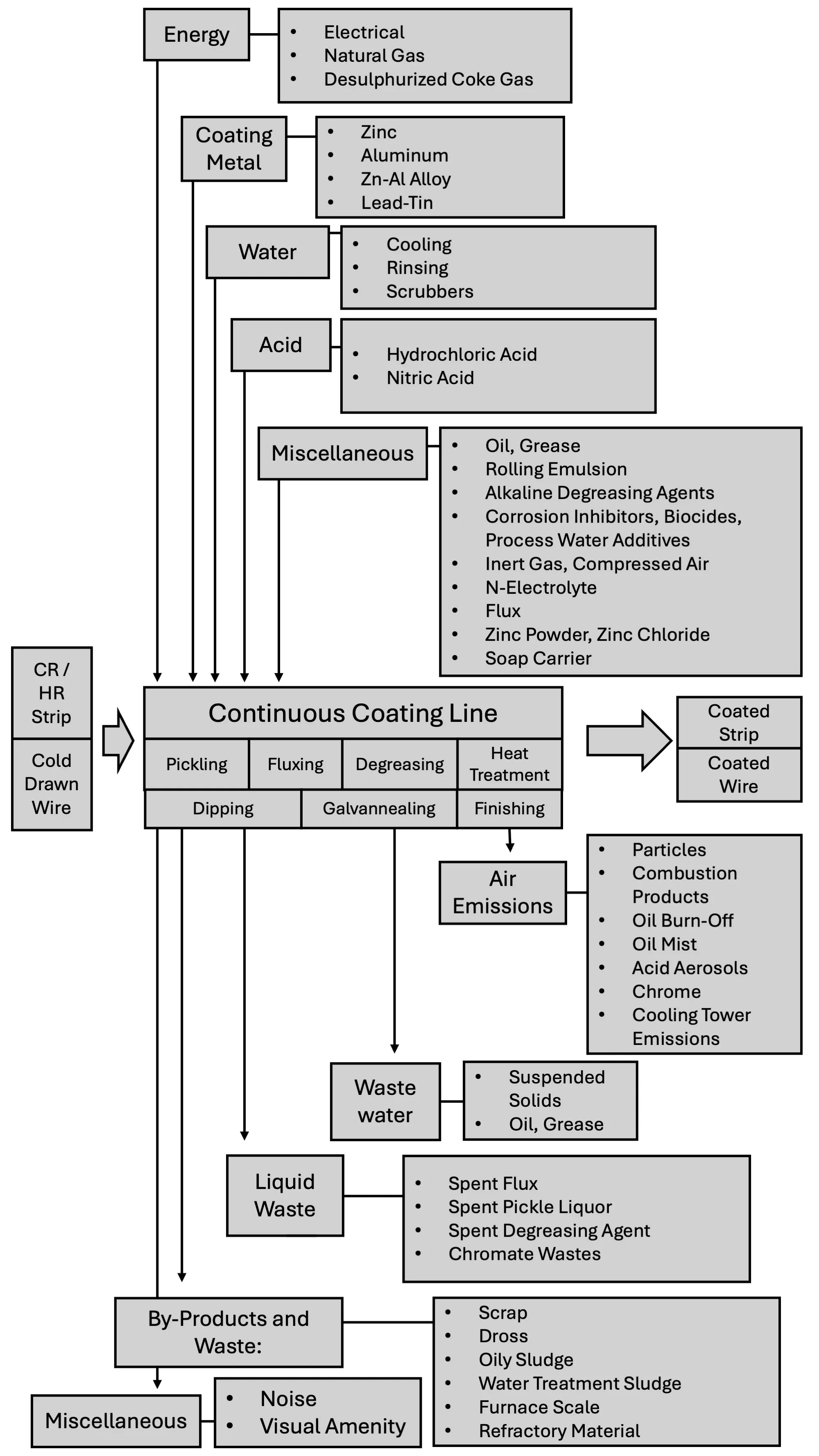
Hot-dip galvanizing is a corrosion protection process in which iron and steel fabrications are coated with zinc to prevent corrosion. Continuous hot-dip coating and batch hot-dip galvanizing are two distinct methods for applying zinc coatings to steel. While continuous hot-dip coating (pre-galvanizing) applies a thinner zinc layer to steel coils before fabrication, hot-dip galvanizing immerses fabricated steel into molten zinc, creating a thicker, more durable coating ideal for harsh outdoor conditions.
Batch hot-dip galvanizing is commonly outsourced to specialized service providers.
The sequence of operations in a batch galvanizing plant typically includes the following process steps:
- Degreasing: Removal of organic contaminants such as dirt, grease, and oil from the metal surface;
- Pickling: Elimination of mill scale and rust by immersing the steel in an acidic solution;
- Fluxing: Application of a flux solution, often zinc ammonium chloride, to remove any remaining oxides and prevent oxidation before immersion in molten zinc;
- Galvanising (melt metal coating): Immersion of the prepared steel into a bath of molten zinc, maintained at approximately 450°C, facilitating a metallurgical reaction between zinc and iron to form zinc-iron alloy layers;
- Finishing: After galvanizing, the steel is cooled, either by quenching in water or by exposure to ambient air, followed by a thorough inspection to ensure coating quality and adherence to specifications.
See the example above for cold rolling (Cold Rolling Mill_With Outsourced Galvanizing).
In the fastener manufacturing industry, carbon dioxide (CO₂) emissions originate from three key sources:
- Electricity consumption: Many manufacturing processes, including machining, assembly, and surface treatments, rely heavily on electricity. The CO₂ emissions associated with this electricity will depend on the energy mix of the grid.
- Fuel Combustion: In many cases, fasteners undergo heat treatments, such as annealing or tempering, which affect their microstructure and thus their physical properties. These processes may utilize furnaces powered by natural gas or other fossil fuels, which generate CO₂ from the combustion.
- Precursor goods: The most significant portion of CO₂ emissions stems from the embedded emissions of the steel products used as inputs (precursors). These embedded emissions encompass the CO₂ emissions released during upstream processes involved in producing crude steel before conversion into finished products.
The diagram below shows the example of an installation producing 1,500 tonnes of bolts and nuts:
Please note that these values are purely for explanatory and illustrative purposes, as each factory will have its unique design
Inputs
CBAM Goods (Relevant Precursors):
- External Precursor:
- Wire rods: 1,570 tonnes
- Direct embedded emissions: 3,108.60 tCO2e
- Indirect embedded emissions: 109.90 tCO2e
Fuels:
- Natural gas: 75,450 m3 --> 480,189.60 tCO2e
Electricity:
- Grid: 1,500 MWh → 342.45 tCO2e (indirect emissions)
Resulting in the following direct and indirect specific embedded emissions (SEE):
- Direct: 2.1751 tCO2e/tonne
- Indirect: 0.3016 tCO2e/tonne
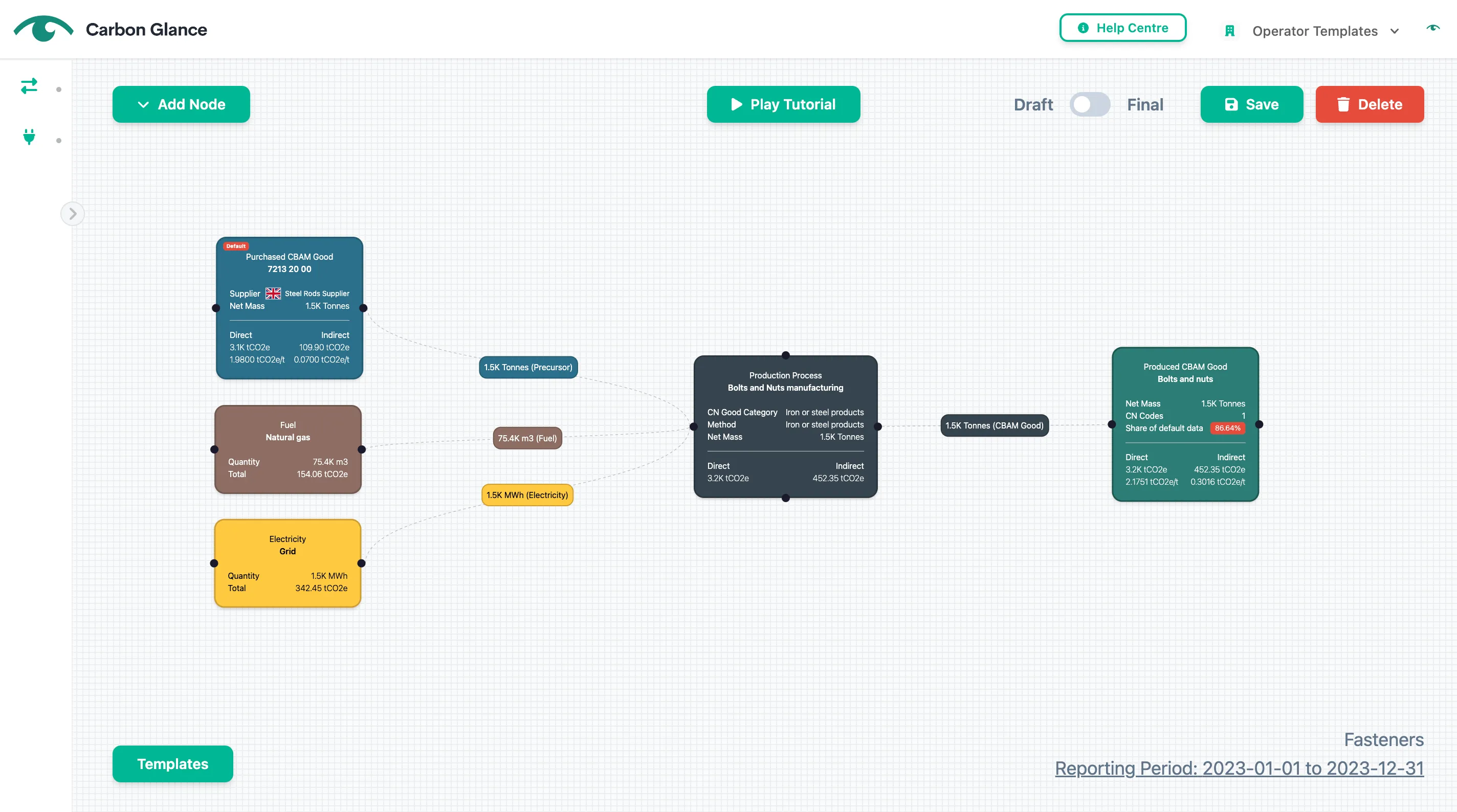
The diagram below also shows the example of an installation producing 1,500 tonnes of bolts and nuts. However, the installation outsources heat treatment (annealing) to an external factory.
According to the EU CBAM, if some processes occur in secondary installations or are outsourced to other companies and the goods are subsequently returned to the primary installation, they may be treated as if they happen in the primary installation.
Please note that these values are purely for explanatory and illustrative purposes, as each factory will have its unique design
Inputs
CBAM Goods (Relevant Precursors):
- External Precursor:
- Wire rods: 1,570 tonnes
- Direct embedded emissions: 3,108.60 tCO2e
- Indirect embedded emissions: 109.90 tCO2e
Fuels (the quantity of fuel used should be provided by the operator of the installation where the annealing took place):
- Natural gas: 75,450 m3 --> 480,189.60 tCO2e
Electricity:
- Internal: Grid: 800 MWh → 182.64 tCO2e (indirect emissions)
- External (the electricity consumed by the factory where the annealing took place, which needs to be collected from that operator's plant): 700 MWh --> 159.81 tCO2e (indirect emissions)
IMPORTANT: If the outsourced process occurs in a different country, ensure you manually enter the electricity emission factor for the secondary installation, as the provided value corresponds to the primary installation's country.
Resulting in the following direct and indirect specific embedded emissions (SEE):
- Direct: 2.1751 tCO2e/tonne
- Indirect: 0.3016 tCO2e/tonne
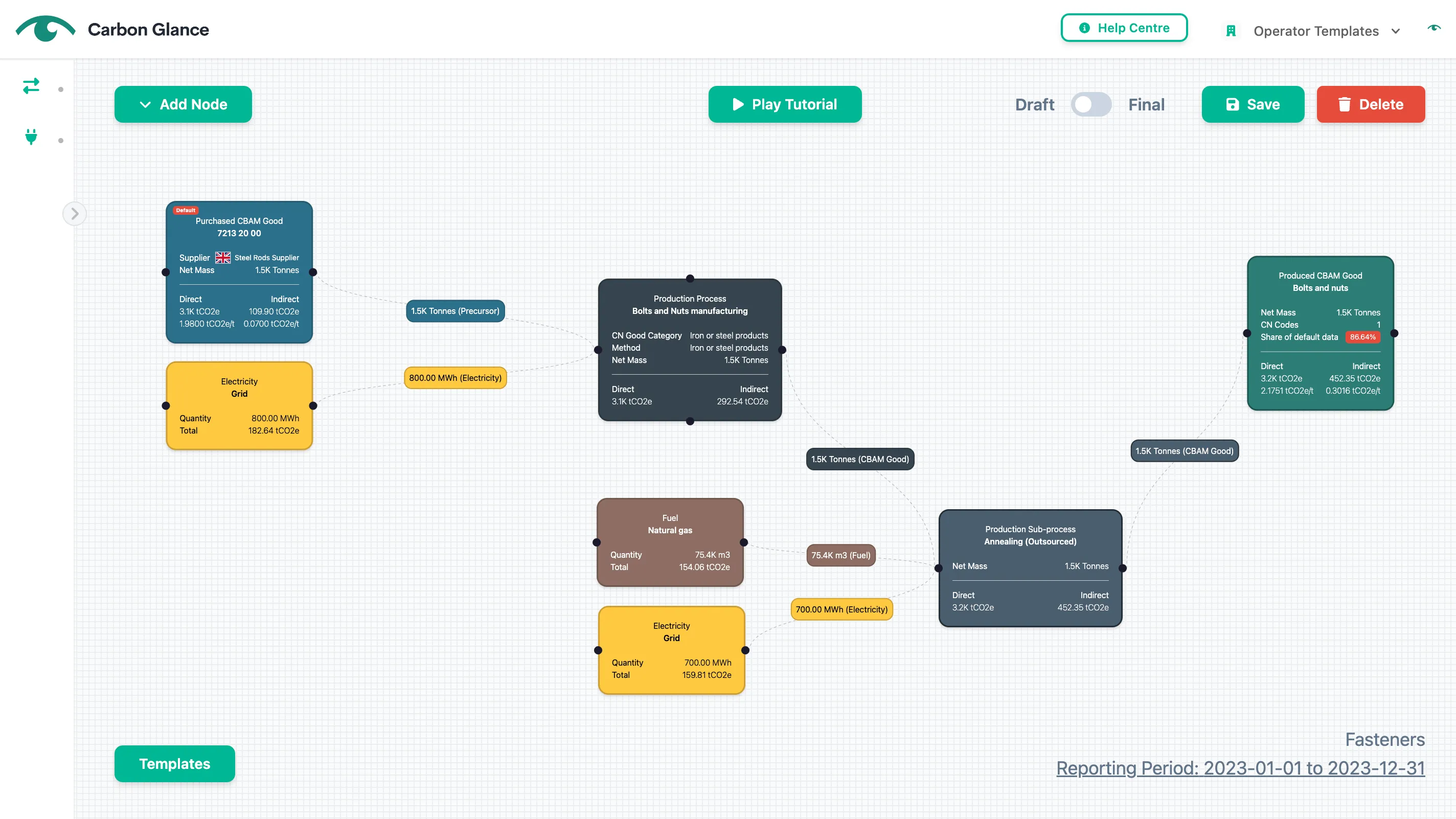